REDLAB: Research in Education and Design


Contact Us:
» Follow us on Twitter: @stanfordREDlab
» REDlab on Facebook
- Stanford University
- School of Education
- 485 Lasuen Mall
- Stanford, CA 94305-3096

- publications and resources
- news and events
Research in Education & Design
©Stanford University. Stanford, CA 94305 (650) 723-2300
Information
- Author Services
Initiatives
You are accessing a machine-readable page. In order to be human-readable, please install an RSS reader.
All articles published by MDPI are made immediately available worldwide under an open access license. No special permission is required to reuse all or part of the article published by MDPI, including figures and tables. For articles published under an open access Creative Common CC BY license, any part of the article may be reused without permission provided that the original article is clearly cited. For more information, please refer to https://www.mdpi.com/openaccess .
Feature papers represent the most advanced research with significant potential for high impact in the field. A Feature Paper should be a substantial original Article that involves several techniques or approaches, provides an outlook for future research directions and describes possible research applications.
Feature papers are submitted upon individual invitation or recommendation by the scientific editors and must receive positive feedback from the reviewers.
Editor’s Choice articles are based on recommendations by the scientific editors of MDPI journals from around the world. Editors select a small number of articles recently published in the journal that they believe will be particularly interesting to readers, or important in the respective research area. The aim is to provide a snapshot of some of the most exciting work published in the various research areas of the journal.
Original Submission Date Received: .
- Active Journals
- Find a Journal
- Proceedings Series
- For Authors
- For Reviewers
- For Editors
- For Librarians
- For Publishers
- For Societies
- For Conference Organizers
- Open Access Policy
- Institutional Open Access Program
- Special Issues Guidelines
- Editorial Process
- Research and Publication Ethics
- Article Processing Charges
- Testimonials
- Preprints.org
- SciProfiles
- Encyclopedia
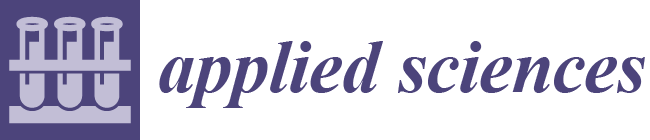
Article Menu
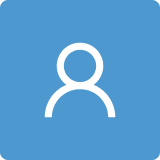
- Subscribe SciFeed
- Recommended Articles
- Google Scholar
- on Google Scholar
- Table of Contents
Find support for a specific problem in the support section of our website.
Please let us know what you think of our products and services.
Visit our dedicated information section to learn more about MDPI.
JSmol Viewer
A systematic review on design thinking integrated learning in k-12 education.
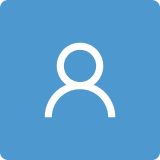
1. Introduction
2.1. keyword search, 2.2. snowball approach, 3.1. what is the current status of dtil in k-12 education, 3.1.1. distribution of articles on design thinking over time, 3.1.2. sample group level, size, and duration, 3.2. what kind of curriculum domains are taught in dtil, 3.2.1. distribution of studies on curriculums, 3.2.2. design thinking model implemented in the empirical studies, 3.3. how to evaluate students’ learning in dtil, 3.3.1. dependent variables, 3.3.2. evaluation instruments, 3.4. what intervention approaches were employed in dtil, 3.4.1. study design, 3.4.2. course type, 3.4.3. grouping, 3.4.4. design thinking task/challenge, 3.4.5. design tools and materials, 4. discussion, 4.1. the current status of dtil, 4.1.1. research trend, 4.1.2. educational level, 4.1.3. samples and duration, 4.2. curriculum domain in dtil, 4.2.1. curriculum, 4.2.2. design thinking model, 4.3. learning evaluation in dtil, 4.3.1. dependent variables, 4.3.2. evaluation instruments, 4.4. interventions in dtil, 4.4.1. study design, 4.4.2. course type, 4.4.3. grouping, 4.4.4. design thinking task/challenge, 4.4.5. learning tools and materials, 5. conclusions and implication, 6. limitations, author contributions, institutional review board statement, informed consent statement, data availability statement, conflicts of interest.
Paper | Sample Size | Educational Level | Duration | Curriculum | Design Thinking Model (Competence) |
---|---|---|---|---|---|
Aflatoony, Wakkary, Neustaedter (2018) [ ] | 39 | high school | 9 weeks | design and technology | A five-step design process including empathise, define, ideate, prototype and test |
Aranda, Lie, Guzey (2020) [ ] | 26 | middle school | 6 days | science | Stage 1: plan a design: cognitive memory, divergent thinking, evaluative thinking. Stage 2: redesign: evaluative thinking. Stage 3: communicate to the client: cognitive memory, divergent thinking |
Blom and Bogaers (2020) [ ] | 18 | middle school | 2 h | technology | N/A |
Carroll et al. (2010) [ ] | 24 | middle school | 3 weeks | geography | The components of the design thinking process include the following: understand, observe, point of view, ideate, prototype, test |
Chin et al. (2019) [ ] | 197 | middle school | 5 weeks | multidisciplinary | N/A |
Christensen et al. (2019) [ ] | 246 | middle school | 2 years | design | Six phases: (1) design problem; (2) field studies; (3) ideation; (4) fabrication; (5) argumentation; (6) reflection |
Cutumisu, Chin, Schwartz (2019) [ ] | 97 | middle school | N/A | multidisciplinary | N/A |
Cutumisu, Schwartz, Lou (2020) [ ] | 80 | middle school | 5 weeks | multidisciplinary | N/A |
Derler et al. (2020) [ ] | 117 | high school | 2 years | multidisciplinary | Three phases: (1) exploration, (2) product ideation, and (3) product prototyping and optimisation |
English (2019) [ ] | 34 | elementary school | N/A | STEM | The inclusion of design processes involved students in learning through design involving planning, sketching, and testing |
Fan, Yu, Lou (2018) [ ] | 103 | high school | 15 weeks | technology and engineering | Clarify problems and constraints, collect information, develop possible solutions, predictive analysis, selection solutions, modelling, and testing, evaluating and revising, optimization |
Fleer (2021) [ ] | 13 | kindergarten | 7 weeks | engineering | Designing, making, appraising |
Forbes et al. (2021) [ ] | 576 | Kindergarten and Primary School | N/A | STEM | Five phases of discovery, interpretation, ideation, experimentation, and evolution to scaffold the ‘design process’ (The IDEO model) |
Gennari, Melonio, Rizvi (2021) [ ] | 8 | middle school | N/A | technology | (1) exploration and familiarisation, (2) ideation and conceptualisation, (3) programming and prototyping |
Gomoll et al. (2018) [ ] | 16 | middle school | 5 weeks | robotics | Ask questions (define problem), imagine (brainstorm ideas), collect information,develop, and test solutions, improve (how did this work? How can we make it better?) |
Guzey and Jung (2021) [ ] | 27 | middle school | 15 days | science | Cognitive memory, convergent thinking, divergent thinking, evaluative thinking |
Kelley and Sung (2017) [ ] | 91 | elementary school | 1 year | science | N/A |
Kelley, Capobianco, Kaluf (2015) [ ] | 21 | elementary school | 1–2 weeks | science | Cognitive processes identified by Halfin’s (1973) study of high-level designers: Analysing, computing, defining problem(s), designing, interpreting data, modelling, predicting, questions/hypotheses, testing. |
Kijima and Sun (2021) [ ] | 26 | middle school | 3 days | STEAM | Five iterative stages (Stanford d.school), including: empathise, define (capture needs), ideate (brainstorm solutions), prototype and test (seek feedback) |
Kijima, Yang-Yoshihara, Maekawa (2021) [ ] | 97 | middle and high school | 3 days | STEAM | Five stages of—from empathy-building to needs—finding, brainstorming, prototyping, and testing |
Kim, Seo, Kim, (2022) [ ] | 28 | elementary school | 3 weeks | engineering | Explore, empathize, ideate, create, test |
Ladachart et al. (2021) [ ] | 38 | middle school | 4 weeks | STEM | Six aspects of design thinking, namely (1) collaboratively working with diversity, (2) being confident and optimistic to use creativity, (3) orientation to learning by making and testing, (4) mindfulness to process and impacts on others, (5) being comfortable with uncertainty and risks, and (6) human-centeredness |
Leinonen et al. (2020) [ ] | 64 | elementary school | 2 months | multidisciplinary | N/A |
Lin et al. (2020) [ ] | 62 | middle school | 7 weeks | technology | Three phases: Inspiration: real-world problem, ideation: design scheme, implementation: digital work |
Lin, Chang, Li (2020) [ ] | 169 | middle school | 8 weeks | engineering | Five stages of engineering design thinking (empathize, define, ideate, prototype, and test) |
Marks and Chase (2019) [ ] | 78 | middle school | 4 weeks | science | Iterative make-test-think process |
Marks and Chase (2019) [ ] | 89 | middle school | 3 weeks | science | Iterative make-test-think process |
Mentzer, Becker, Sutton (2015) [ ] | 59 | high school | 3 h | engineering | 8 stages: problem definition,information gathering, idea generation, modelling, feasibility, evaluation, decision making, communication |
Mentzer, Huffman, Thayer (2014) [ ] | 20 | high school | 3 h | engineering | Problem definition, gathering information, generating ideas, modelling, feasibility, evaluation, decision, communication |
Nichols et al. (2021) [ ] | 159 | elementary school | 12 weeks | science | Defining, designing, producing, evaluating |
Parikh, Maddulety, Meadows (2020) [ ] | 70 | middle school | 5 months | N/A | N/A |
Rao, Puranam, Singh (2022) [ ] | 195 | middle school | 4 days | science | Four stages called ‘feel’, ‘imagine’, ‘do’ and ‘share’ |
Simeon, Samsudin, Yakob (2022) [ ] | 89 | high school | 3 months | science | The five-stage model proposed by the Hasso Plattner Institute of Design at Stanford: empathy, define (the problem), ideate, prototype, and test |
Sung and Kelley (2019) [ ] | 27 | elementary school | 1 year | science | Identify problem, share and develop a plan, create, and test, communicate results and gather feedback, improve and retest |
Tsai and Wang (2021) [ ] | 350 | middle school | N/A | STEAM | Four phases: empathize, define, ideate and prototype |
Van Mechelen et al. (2019) [ ] | 49 | elementary school | 1 day | N/A | The Collaborative Design Thinking (CoDeT): introduction, sensitizing, scaffolding collaboration, defining a design goal, reflection on collaboration, ideation, grouping and selection, elaboration through making, presentation and peer jury, iteration or wrap up |
Wendell, Wright, Paugh (2017) [ ] | N/A | elementary school | 1 day | science | Reflective decision making: articulate multiple solutions, evaluate pros and cons, intentionally select solution, retell performance of solution, analyse solution according to specific evidence, purposefully choose improvements |
Won et al. (2015) [ ] | 44 | middle school | N/A | STEM | Articulation of the learning phenomenon, design, data collection, actual construction, redesign |
Yalcin and Erden (2021) [ ] | 39 | kindergarten | 8 weeks | STEM | Five stages used by the Hasso Platter Institute at Stanford: Empathize, Define, Ideate, Prototype, Test |
Yu, Wu, Fan (2020) [ ] | 613 | high school | 16 weeks | engineering | Observing, predicting, creating, analysing, and evaluating |
Zhang et al. (2022) [ ] | 30 | middle school | 3 months | Design & Research | Empathize Design User (EDU), Define Design Problem (DDP), Ideate Design Solution (IDS), Make Prototype (MP), Test Prototype (TP) |
Zhou et al. (2017) [ ] | 24 | middle school | 2 weeks | science and engineering | Nine coding categories: sketching, prototyping, design goals, inference/predictions about design, generate design ideas, design of structure, design of system/process, materials, and collaboration |
Zhou et al. (2021) [ ] | 27 | middle school | 2 weeks | engineering | Design cycle of planning, building, and testing |
Zupan, Cankar, Cankar (2018) [ ] | 146 | elementary school | 17.5 weeks | N/A | The process was divided into five interrelated phases: understanding and defining the problem, observation, ideation, prototyping and testing, implementation |
Paper | Course Type | Task/Challenge | Tools and Materials | Grouping | Study Design | Study Type | Dependent Variable | Measurement Instrument |
---|---|---|---|---|---|---|---|---|
Aflatoony, Wakkary, Neustaedter (2018) [ ] | formal | To use design to change their communities | N/A | 3–4 | Non-experimental: design thinking based pedagogy in the context of interaction design | O X O | Design thinking | Observation, survey, portfolio |
Aranda, Lie, Guzey (2020) [ ] | formal | Design a process to both prevent and test for cross-pollination of non-GMO fields from GMO fields. (Genetically Modified Organisms, GMOs) | N/A | 4 | Non-experimental: engineering design as a tool to improve student science learning | X O | Skill | Protocol analysis |
Blom and Bogaers (2020) [ ] | N/A | Design a heat retaining food container for street food vendors at a taxi depot | Basic stationary items, including pens, pencils, safety rulers, post-it notes, coloured pencils, paper, paper clips, felt-tip pens and highlighters. | 3 | Non-experimental: STEM design task | X O | Design thinking | Protocol analysis, video recording |
Carroll et al. (2010) [ ] | formal | To identify and redesign systems that existed at the school | N/A | 4–5 | Non-experimental: introducing students both to the design process and to systems in geography | X O | Design thinking, emotional/social aspect | Journal, audio recording, video recording, portfolio, interview |
Chin et al. (2019) [ ] | formal | Design digital posters | Computer design games | 1 | Experimental: EG1: Feedback design-thinking strategies treatment; EG2: Explore design-thinking strategies treatment | R O X1 O R O X2 O | Design thinking | Test |
Christensen et al. (2019) [ ] | formal | To create a secure environment for the elderly without taking away their freedom | Digital technology | N/A | Quasi-experimental: EG: students who had already received design education in their school (FabLab group); CG: without intervention | X1 O X2 O | Design thinking, emotional/social aspect | Questionnaire |
Cutumisu, Chin, Schwartz (2019) [ ] | formal | Design digital posters | Computer design games | 1 | Non-experimental: digital poster design game (Posterlet) | X O | Design thinking, subject learning performance | Test, design work evaluation |
Cutumisu, Schwartz, Lou (2020) [ ] | formal | Design digital posters | Computer design games | 1 | Non-experimental: digital poster design game (Posterlet) | O X O | Design thinking | Test |
Derler et al. (2020) [ ] | formal | To develop sustainable food products for peer group | N/A | Group | Non-experimental: Project-Based Learning focused on the development of sustainable food products | X O | Design thinking | Portfolio, journal, survey, interview |
English (2019) [ ] | formal | Design and construct shoes | N/A | 3 | Non-experimental: problem solving activities (shoes design) | X O | Design thinking | Portfolio, protocol analysis |
Fan, Yu, Lou (2018) [ ] | formal | To design a movable toy with various mechanical structure types | Physical and 3D virtual simulation models, LEGO | N/A | Non-experimental: project-based engineering design program | O X O | Subject learning performance, design thinking, emotional/social aspect | Test, questionnaire, design work evaluation, survey, observation |
Fleer (2021) [ ] | formal | Design castle according to an imaginary engineering situation of Sherwood forest | N/A | Individual and collective | Non-experimental: designerly play | X O | Design thinking | Observation |
Forbes et al. (2021) [ ] | formal | 3D design and printing: Floatable boats, shadow puppets, Headphone cable holders, Spinning tops, Playground sculptures, Habitat for hermit crabs, Herb markers, Designing keyrings, Bag tags | 3D design and printing technologies (Ipad and 3D design software and print device) | N/A | Non-experimental: STEM-focussed curricula in 3D technology based makerspace | X O | Subject learning performance, design thinking, skill, emotional/social aspect | Interview, survey, journal, observation |
Gennari, Melonio, Rizvi (2021) [ ] | N/A | Generate smart-things ideas for an outdoor park environment | Card-based toolkits, microelectronics components: Raspberry Pi, Google’s Design Sprint Kit | N/A | Non-experimental: IoT design workshop | X O | Design thinking, emotional/social aspect | Portfolio, questionnaire, observation, interview |
Gomoll et al. (2018) [ ] | formal | Design a robot that served a need in their local environment and allowed remote peers to explore their local spaces | A mobile telepresence robot that we called KT, controlled over the Internet through a web interface | 4 | Non-experimental: human-centred robotics curriculum | X O | Design thinking | Portfolio, audio recording, video recording |
Guzey and Jung (2021) [ ] | formal | Design a water filter system for the city’s wastewater management plant to help prevent the pollution of a local river | N/A | 3–4 | Non-experimental: engineering design task in teams | O X O | Skill, subject learning performance | Audio recording, protocol analysis, test |
Kelley and Sung (2017) [ ] | formal | 3 engineering design activities: Musical Instrument, Simple Machines, and bio-inspired flower | N/A | 2–4 | Quasi-experimental: EG1: pretreatment on basic engineering design sketching strategies before the three design activities; EG2: delayed treatment before the third design activity | X1 O X2 O | Design thinking | Design work evaluation |
Kelley, Capobianco, Kaluf (2015) [ ] | formal | To work in teams to build a prototype for a prosthetic leg to function like a human leg joint and strike the ball; paper football kicker | N/A | 3 | Non-experimental: engineering design activity | X O | Design thinking | Protocol analysis, video recording |
Kijima and Sun (2021) [ ] | N/A | Work revolved around interviewing senior citizens and creating prototypes that met their needs on the background of Japan’s aging society | N/A | N/A | Non-experimental: design thinking workshop | O X O | Emotional/social aspect | Survey |
Kijima, Yang-Yoshihara, Maekawa (2021) [ ] | N/A | Design local solutions addressing global issues | Using basic prototyping materials such as recycled plastic bottles and cardboards, glue, tapes, scissors, | 4 | Non-experimental: design thinking and STEAM workshop | O X O | Emotional/social aspect | Questionnaire, interview |
Kim, Seo, Kim, (2022) [ ] | formal | Reading the narrative content of the books and solving the engineering problems presented in the books, and finally rewrite the story | COBL-S (Arduino Leonardo-based device, supported programming language was developed based on Scratch and app inventor) | 3–4 | Quasi-experimental: EG: Class activities according to the NE-Maker instructional model; CG: Normal software education class according to the textbook. | O X1 O O X2 O | Emotional/social aspect | Questionnaire, journal, interview |
Ladachart et al. (2021) [ ] | formal | Reverse engineering project: to design a bimetal thermostat | A dissected bimetal thermostat, metal, tape, and scissors | 3 | Non-experimental: design-based reverse engineering | O X O | Emotional/social aspect | Questionnaire, video recording, protocol analysis |
Leinonen et al. (2020) [ ] | formal | 3D model design and 3D artefact printing | 3D model design by Tinkercad software and 3D artefact printing by Ultimaker printer | group | Non-experimental: 3D design and printing activities | X O | Subject learning performance, skill, design thinking | Observation, interview, questionnaire, portfolio |
Lin et al. (2020) [ ] | formal | Design digital documents for new year party (e.g., to make posters for party promotion) | WPS Writer | group | Quasi-experiment: EG: using the design thinking approach (class a: project); CG: using traditional teaching methods (class b, according to the textbook) | O X1 O O X2 O | Subject learning performance | Design work evaluation |
Lin, Chang, Li (2020) [ ] | formal | Design an electric model vehicle capable of automatically avoiding obstacles was developed | The experimental group experienced design teaching with VR devices used as teaching tools | N/A | Experimental: EG: engineering design teaching with VR CG: conventional engineering design teaching | R O X1 O R O X2 O | Design thinking | Survey, design work evaluation |
Marks and Chase (2019) [ ] | formal | Drop challenge, playground challenge, and a post-design challenge (the boat challenge) | N/A | N/A | Experimental: EG: iterative prototyping (Prototype); CG: content-focused design (Content) | R O X1 O R O X2 O | Design thinking, emotional/social aspect | Test, survey, design work evaluation |
Marks and Chase (2019) [ ] | formal | Base-line tower design task,drop challenge, playground challenge, and a post-design challenge (the boat challenge) | N/A | N/A | Experimental: EG: design thinking intervention focused on effective iterative prototyping (Prototype); CG: content-focused intervention (Content) | R O X1 O R O X2 O | Design thinking, emotional/social aspect | Test, survey, design work evaluation |
Mentzer, Becker, Sutton (2015) [ ] | formal | To design a playground | A calculator, ruler, a small note pad, graph paper, white paper, pencil, highlighter, sticky notes, and a piece of paper identifying the design task were placed on the table before the student entered the room | N/A | Quasi-experimental: EG1: high school freshmen starting the sequence of engineering courses; EG2: high school seniors who had taken multiple engineering courses; CG: engineering experts | X1 O X2 O X3 O | Design thinking | Audio recording, video recording, protocol analysis, portfolio |
Mentzer, Huffman, Thayer (2014) [ ] | formal | Playground design | N/A | 1 | Non-experimental: Engineering design challenge | X O | Design thinking, Emotional/social aspect | Observation, protocol Analysis, video recording, audio recording, portfolio, interview, survey |
Nichols et al. (2021) [ ] | formal | To design and construct a device that is engineered to provide electricity to a third world community scenario | Materials like LED, water | 2–4 | Quasi-experimental: EG: design task embedded in an inquiry science unit and a community of inquiry (CoI); CG: design task embedded in an inquiry science unit (Non-CoI) | O X1 O O X2 O | Design thinking, subject learning performance | Protocol analysis, video recording, test, interview |
Parikh, Maddulety, Meadows (2020) [ ] | informal | Design prototypes for solving a Design Thinking challenge | N/A | 5–6 | Quasi-experiment: EG: Design Thinking training spread over two action research cycles; CG: received no intervention | O X1 O X2 O O X3 O X4 O | Skill | Portfolio, test |
Rao, Puranam, Singh (2022) [ ] | informal | Three key design thinking exercises: ’Bag Exercise’, ’Cartographer’, ‘Be a Detective’ | N/A | N/A | Experimental: EG: design thinking training programme; CG: usual hands-on science education curriculum | R X1 O R X2 O | Skill | Test |
Simeon, Samsudin, Yakob (2022) [ ] | informal | Zip line delivery challenge,truss bridge challenge | N/A | N/A | Non-experimental: STEM- Design thinking modules | O X O | Subject learning performance | Test |
Sung and Kelley (2019) [ ] | formal | Design a Doggie Door Alarm | Normal design tools, such as paper, pencil | 3 | Non-experimental: engineering design activity for science learning | X O | Design thinking | Audio recording, video recording, protocol analysis |
Tsai and Wang (2021) [ ] | formal | Design a robot for solving some problems related to natural science or ecological environmental issues | N/A | N/A | Non-experimental: project-based STEAM curriculum | X O | Emotional/social aspect | Questionnaire |
Van Mechelen et al. (2019) [ ] | formal | The design theme on preventing bullying in the social context of the class | N/A | 4–6 | Non-experimental: design activities on the theme of preventing bullying in the social context of the class | X O | Emotional/social aspect, design thinking | Observation, portfolio, design work evaluation |
Wendell, Wright, Paugh (2017) [ ] | formal | Design water filters, bridges, circuits in, maglev vehicles and windmills, and pollinators and knee braces | N/A | 2–3 | Non-experimental: engineering design tasks | X O | Design thinking | Video recording, portfolio, protocol analysis |
Won et al. (2015) [ ] | informal | Design of lights powered through motion | Social media technologies | N/A | Non-experimental: integrating learning technologies such as social networking forum (SNF) into design-based learning activities | X O | Design thinking | Survey, portfolio |
Yalcin and Erden (2021) [ ] | formal | Design thinking STEM activities | N/A | 4 | Experimental: EG: design thinking STEM activities CG: non-STEM activities | R O X1 O R O X2 O | Skill | Survey, journal |
Yu, Wu, Fan (2020) [ ] | formal | Design mechanical toy | The design stage used computer-aided design to create a three-dimensional model of the toy | N/A | Non-experimental: engineering project | X O | Subject learning performance, design thinking, skill | Questionnaire, design work evaluation, portfolio |
Zhang et al. (2022) [ ] | formal | Design an escape room for the local fire department to allow participants to playfully and interactively improve awareness of fire safety in and around the house | N/A | 3–4 | Non-experimental: DBL (design-based learning) activities | X O | Design thinking, emotional/social aspect | Questionnaire, observation, interview |
Zhou et al. (2017) [ ] | informal | A total of five toy design activities | N/A | 3–4 | Non-experimental: toy design workshop | O X O | Emotional/social aspect, design thinking | Questionnaire, survey |
Zhou et al. (2021) [ ] | informal | Marshmallow tower activity and the trebuchet design activity | N/A | 3–4 | Non-experimental: design workshop | X O | Design thinking | Observation, design work evaluation |
Zupan, Cankar, Cankar (2018) [ ] | formal | Identify and define a local or social problem that could be solved with a new product, service, or other solution | N/A | N/A | Non-experimental: use the design thinking method to develop the entrepreneurial mindset | X O | Emotional/social aspect | Interview, observation |
- Kimbell, L. Rethinking Design Thinking: Part I. Des. Cult. 2011 , 3 , 285–306. [ Google Scholar ] [ CrossRef ]
- Micheli, P.; Wilner, S.J.; Bhatti, S.H.; Mura, M.; Beverland, M.B. Doing design thinking: Conceptual review, synthesis, and research agenda. J. Prod. Innov. Manag. 2019 , 36 , 124–148. [ Google Scholar ] [ CrossRef ]
- Simon, H.A. The Sciences of the Artificial ; MIT Press: Cambridge, MA, USA, 1969. [ Google Scholar ]
- Cross, N. Expertise in design: An overview. Des. Stud. 2004 , 25 , 427–441. [ Google Scholar ] [ CrossRef ]
- Jonassen, D.H. Toward a design theory of problem solving. Educ. Technol. Res. Dev. 2000 , 48 , 63–85. [ Google Scholar ] [ CrossRef ]
- Dorst, K. The core of ‘design thinking’and its application. Des. Stud. 2011 , 32 , 521–532. [ Google Scholar ] [ CrossRef ]
- Brown, T. Design thinking. Harv. Bus. Rev. 2008 , 86 , 84–92. Available online: https://pubmed.ncbi.nlm.nih.gov/18605031/ (accessed on 9 June 2022).
- Kelley, T.; Kelley, D. Creative Confidence: Unleashing the Creative Potential Within Us All ; Crown Business: New York, NY, USA, 2013. [ Google Scholar ]
- Martin, R.; Martin, R.L. The Design of Business: Why Design Thinking is the Next Competitive Advantage ; Harvard Business Press: Boston, MA, USA, 2009. [ Google Scholar ]
- Brenner, W.; Uebernickel, F.; Abrell, T. Design thinking as mindset, process, and toolbox. In Design Thinking for Innovation ; Springer: Berlin, Germany, 2016; pp. 3–21. [ Google Scholar ] [ CrossRef ]
- Rusmann, A.; Ejsing-Duun, S. When design thinking goes to school: A literature review of design competences for the K-12 level. Int. J. Technol. Des. Educ. 2021 . [ Google Scholar ] [ CrossRef ]
- Zhang, F.; Markopoulos, P.; Bekker, T. Children’s Emotions in Design-Based Learning: A Systematic Review. J. Sci. Educ. Technol. 2020 , 29 , 459–481. [ Google Scholar ] [ CrossRef ]
- Zhou, D.; Gomez, R.; Wright, N.; Rittenbruch, M.; Davis, J. A design-led conceptual framework for developing school integrated STEM programs: The Australian context. Int. J. Technol. Des. Educ. 2020 , 32 , 383–411. [ Google Scholar ] [ CrossRef ]
- International Technology and Engineering Educators Association. Standards for Technological and Engineering Literacy: The Role of Technology and Engineering in STEM Education. Available online: https://www.iteea.org/STEL.aspx (accessed on 9 June 2022).
- Johansson-Sköldberg, U.; Woodilla, J.; Çetinkaya, M. Design thinking: Past, present and possible futures. Creat. Innov. Manag. 2013 , 22 , 121–146. [ Google Scholar ] [ CrossRef ]
- Razzouk, R.; Shute, V. What is design thinking and why is it important? Rev. Educ. Res. 2012 , 82 , 330–348. [ Google Scholar ] [ CrossRef ]
- Hasso Plattner Institute of Design at Stanford University. Design Thinking Bootleg. Available online: https://dschool.stanford.edu/resources/design-thinking-bootleg (accessed on 9 June 2022).
- IDEO. Design Thinking for Educators Toolkit (2nd ed.). Available online: https://designthinkingforeducators.com/toolkit/ (accessed on 9 June 2022).
- Design Council. The Double Diamond: A Universally Accepted Depiction of the Design Process. Available online: https://www.designcouncil.org.uk/our-work/news-opinion/double-diamond-universally-accepted-depiction-design-process/ (accessed on 9 June 2022).
- Burghardt, M.D.; Hacker, M. Informed design: A contemporary approach to design pedagogy as the core process in technology. Technol. Teach. 2004 , 64 , 6–8. [ Google Scholar ]
- English, L.D. Learning while designing in a fourth-grade integrated STEM problem. Int. J. Technol. Des. Educ. 2018 , 29 , 1011–1032. [ Google Scholar ] [ CrossRef ]
- Chase, C.C.; Malkiewich, L.; Kumar, A.S. Learning to notice science concepts in engineering activities and transfer situations. Sci. Educ. 2018 , 103 , 440–471. [ Google Scholar ] [ CrossRef ]
- Rao, H.; Puranam, P.; Singh, J. Does design thinking training increase creativity? Results from a field experiment with middle-school students. Innovation 2021 , 24 , 315–332. [ Google Scholar ] [ CrossRef ]
- Tranfield, D.; Denyer, D.; Smart, P. Towards a Methodology for Developing Evidence-Informed Management Knowledge by Means of Systematic Review. Br. J. Manag. 2003 , 14 , 207–222. [ Google Scholar ] [ CrossRef ]
- Wohlin, C. Guidelines for snowballing in systematic literature studies and a replication in software engineering. In Proceedings of the 18th international conference on evaluation and assessment in software engineering, London, UK, 13 May 2014; pp. 1–10. [ Google Scholar ] [ CrossRef ]
- Yu, K.C.; Wu, P.H.; Fan, S.C. Structural Relationships among High School Students’ Scientific Knowledge, Critical Thinking, Engineering Design Process, and Design Product. Int. J. Sci. Math. Educ. 2020 , 18 , 1001–1022. [ Google Scholar ] [ CrossRef ]
- Forbes, A.; Falloon, G.; Stevenson, M.; Hatzigianni, M.; Bower, M. An Analysis of the Nature of Young Students’ STEM Learning in 3D Technology-Enhanced Makerspaces. Early Educ. Dev. 2020 , 32 , 172–187. [ Google Scholar ] [ CrossRef ]
- Tsai, M.-J.; Wang, C.-Y. Assessing Young Students’ Design Thinking Disposition and Its Relationship With Computer Programming Self-Efficacy. J. Educ. Comput. Res. 2020 , 59 , 410–428. [ Google Scholar ] [ CrossRef ]
- Yalcin, V.; Erden, S. The Effect of STEM Activities Prepared According to the Design Thinking Model on Preschool Children’s Creativity and Problem-Solving Skills. Think. Ski. Creat. 2021 , 41 , 100864. [ Google Scholar ] [ CrossRef ]
- Zhang, F.; Markopoulos, P.; Bekker, T.; Paule-Ruíz, M.; Schüll, M. Understanding design-based learning context and the associated emotional experience. Int. J. Technol. Des. Educ. 2020 , 32 , 845–882. [ Google Scholar ] [ CrossRef ]
- Lin, H.-C.; Chang, Y.-S.; Li, W.-H. Effects of a virtual reality teaching application on engineering design creativity of boys and girls. Think. Ski. Creat. 2020 , 37 , 100705. [ Google Scholar ] [ CrossRef ]
- Kijima, R.; Sun, K.L. ‘Females Don’t Need to be Reluctant’: Employing Design Thinking to Harness Creative Confidence and Interest in STEAM. Int. J. Art Des. Educ. 2020 , 40 , 66–81. [ Google Scholar ] [ CrossRef ]
- Aflatoony, L.; Wakkary, R.; Neustaedter, C. Becoming a Design Thinker: Assessing the Learning Process of Students in a Secondary Level Design Thinking Course. Int. J. Art Des. Educ. 2017 , 37 , 438–453. [ Google Scholar ] [ CrossRef ]
- Guzey, S.S.; Jung, J.Y. Productive Thinking and Science Learning in Design Teams. Int. J. Sci. Math. Educ. 2021 , 19 , 215–232. [ Google Scholar ] [ CrossRef ]
- Marks, J.; Chase, C.C. Impact of a prototyping intervention on middle school students’ iterative practices and reactions to failure. J. Eng. Educ. 2019 , 108 , 547–573. [ Google Scholar ] [ CrossRef ]
- Nichols, K.; Musofer, R.; Fynes-Clinton, L.; Blundell, R. Design thinking and inquiry behaviours are co-constituted in a community of inquiry middle years’ science classroom context: Empirical evidence for design thinking and pragmatist inquiry interconnections. Int. J. Technol. Des. Educ. 2021 . [ Google Scholar ] [ CrossRef ]
- Simeon, M.I.; Samsudin, M.A.; Yakob, N. Effect of design thinking approach on students’ achievement in some selected physics concepts in the context of STEM learning. Int. J. Technol. Des. Educ. 2020 , 32 , 185–212. [ Google Scholar ] [ CrossRef ]
- Carroll, M.; Goldman, S.; Britos, L.; Koh, J.; Royalty, A.; Hornstein, M. Destination, Imagination and the Fires Within: Design Thinking in a Middle School Classroom. Int. J. Art Des. Educ. 2010 , 29 , 37–53. [ Google Scholar ] [ CrossRef ]
- Zhou, N.; Pereira, N.; George, T.T.; Alperovich, J.; Booth, J.; Chandrasegaran, S.; Tew, J.D.; Kulkarni, D.M.; Ramani, K. The Influence of Toy Design Activities on Middle School Students’ Understanding of the Engineering Design Processes. J. Sci. Educ. Technol. 2017 , 26 , 481–493. [ Google Scholar ] [ CrossRef ]
- Christensen, K.S.; Hjorth, M.; Iversen, O.S.; Smith, R.C. Understanding design literacy in middle-school education: Assessing students’ stances towards inquiry. Int. J. Technol. Des. Educ. 2018 , 29 , 633–654. [ Google Scholar ] [ CrossRef ]
- Mentzer, N.; Huffman, T.; Thayer, H. High school student modeling in the engineering design process. Int. J. Technol. Des. Educ. 2014 , 24 , 293–316. [ Google Scholar ] [ CrossRef ]
- Mentzer, N.; Becker, K.; Sutton, M. Engineering Design Thinking: High School Students’ Performance and Knowledge. J. Eng. Educ. 2015 , 104 , 417–432. [ Google Scholar ] [ CrossRef ]
- Fan, S.C.; Yu, K.C.; Lou, S.J. Why do students present different design objectives in engineering design projects? Int. J. Technol. Des. Educ. 2018 , 28 , 1039–1060. [ Google Scholar ] [ CrossRef ]
- Sung, E.; Kelley, T.R. Identifying design process patterns: A sequential analysis study of design thinking. Int. J. Technol. Des. Educ. 2018 , 29 , 283–302. [ Google Scholar ] [ CrossRef ]
- Zhou, N.; Pereira, N.; Chandrasegaran, S.; George, T.T.; Booth, J.; Ramani, K. Examining Middle School Students’ Engineering Design Processes in a Design Workshop. Res. Sci. Educ. 2019 , 51 (Suppl. 2), 617–646. [ Google Scholar ] [ CrossRef ]
- Blom, N.; Bogaers, A. Using Linkography to investigate students’ thinking and information use during a STEM task. Int. J. Technol. Des. Educ. 2018 , 30 , 1–20. [ Google Scholar ] [ CrossRef ]
- Kelley, T.R.; Sung, E. Sketching by design: Teaching sketching to young learners. Int. J. Technol. Des. Educ. 2017 , 27 , 363–386. [ Google Scholar ] [ CrossRef ]
- Lin, L.; Shadiev, R.; Hwang, W.-Y.; Shen, S. From knowledge and skills to digital works: An application of design thinking in the information technology course. Think. Ski. Creat. 2020 , 36 , 100646. [ Google Scholar ] [ CrossRef ]
- Gennari, R.; Melonio, A.; Rizvi, M. From children’s ideas to prototypes for the internet of things: A case study of cross-generational end-user design. Behav. Inf. Technol. 2021 . [ Google Scholar ] [ CrossRef ]
- Kijima, R.; Yang-Yoshihara, M.; Maekawa, M.S. Using design thinking to cultivate the next generation of female STEAM thinkers. Int. J. STEM Educ. 2021 , 8 , 1–15. [ Google Scholar ] [ CrossRef ]
- Ladachart, L.; Cholsin, J.; Kwanpet, S.; Teerapanpong, R.; Dessi, A.; Phuangsuwan, L.; Phothong, W. Ninth-grade students’ perceptions on the design-thinking mindset in the context of reverse engineering. Int. J. Technol. Des. Educ. 2021. [ CrossRef ]
- Kim, J.-Y.; Seo, J.S.; Kim, K. Development of novel-engineering-based maker education instructional model. Educ. Inf. Technol. 2022 , 27 , 7327–7371. [ Google Scholar ] [ CrossRef ]
- Parikh, C.; Maddulety, K.; Meadows, C. Improving creative ability of base of pyramid (BOP) students in India. Think. Ski. Creativity 2020 , 36 , 100652. [ Google Scholar ] [ CrossRef ]
- Aranda, M.L.; Lie, R.; Guzey, S.S. Productive thinking in middle school science students’ design conversations in a design-based engineering challenge. Int. J. Technol. Des. Educ. 2019 , 30 , 67–81. [ Google Scholar ] [ CrossRef ]
- Derler, H.; Berner, S.; Grach, D.; Posch, A.; Seebacher, U. Project-Based Learning in a Transinstitutional Research Setting: Case Study on the Development of Sustainable Food Products. Sustainability 2019 , 12 , 233. [ Google Scholar ] [ CrossRef ]
- Won, S.G.; Evans, M.A.; Carey, C.; Schnittka, C.G. Youth appropriation of social media for collaborative and facilitated design-based learning. Comput. Hum. Behav. 2015 , 50 , 385–391. [ Google Scholar ] [ CrossRef ]
- Leinonen, T.; Virnes, M.; Hietala, I.; Brinck, J. 3D Printing in the Wild: Adopting Digital Fabrication in Elementary School Education. Int. J. Art Des. Educ. 2020 , 39 , 600–615. [ Google Scholar ] [ CrossRef ]
- Van Mechelen, M.; Laenen, A.; Zaman, B.; Willems, B.; Abeele, V.V. Collaborative Design Thinking (CoDeT): A co-design approach for high child-to-adult ratios. Int. J. Human-Computer Stud. 2019 , 130 , 179–195. [ Google Scholar ] [ CrossRef ]
- Kelley, T.R.; Capobianco, B.M.; Kaluf, K.J. Concurrent think-aloud protocols to assess elementary design students. Int. J. Technol. Des. Educ. 2014 , 25 , 521–540. [ Google Scholar ] [ CrossRef ]
- Cutumisu, M.; Chin, D.B.; Schwartz, D.L. A digital game-based assessment of middle-school and college students’ choices to seek critical feedback and to revise. Br. J. Educ. Technol. 2019 , 50 , 2977–3003. [ Google Scholar ] [ CrossRef ]
- Gomoll, A.; Tolar, E.; Hmelo-Silver, C.E.; Šabanović, S. Designing human-centered robots: The role of constructive failure. Think. Ski. Creat. 2018 , 30 , 90–102. [ Google Scholar ] [ CrossRef ]
- Wendell, K.B.; Wright, C.G.; Paugh, P. Reflective Decision-Making in Elementary Students’ Engineering Design. J. Eng. Educ. 2017 , 106 , 356–397. [ Google Scholar ] [ CrossRef ]
- Trochim, W.M.K.; Donnelly, J.P. Research Methods Knowledge Base (3rd ed.). Available online: http://www.socialresearchmethods.net/kb/ (accessed on 9 June 2022).
- Fleer, M. The genesis of design: Learning about design, learning through design to learning design in play. Int. J. Technol. Des. Educ. 2022 , 32 , 1441–1468. [ Google Scholar ] [ CrossRef ]
- Cutumisu, M.; Schwartz, D.L.; Lou, N.M. The relation between academic achievement and the spontaneous use of design-thinking strategies. Comput. Educ. 2020 , 149 , 103806. [ Google Scholar ] [ CrossRef ]
- Zupan, B.; Cankar, F.; Cankar, S.S. The development of an entrepreneurial mindset in primary education. Eur. J. Educ. 2018 , 53 , 427–439. [ Google Scholar ] [ CrossRef ]
- Buchanan, R. Wicked Problems in Design Thinking. Des. Issues 1992 , 8 , 5–21. [ Google Scholar ] [ CrossRef ]
- Chin, D.B.; Blair, K.P.; Wolf, R.C.; Conlin, L.D.; Cutumisu, M.; Pfaffman, J.; Schwartz, D.L. Educating and Measuring Choice: A Test of the Transfer of Design Thinking in Problem Solving and Learning. J. Learn. Sci. 2019 , 28 , 337–380. [ Google Scholar ] [ CrossRef ]
- Csikszentmihalyi, M. Good Business: Leadership, Flow, and the Making of Meaning ; Viking: New York, NY, USA, 2004. [ Google Scholar ]
- Wells, A. The importance of design thinking for technological literacy: A phenomenological perspective. Int. J. Technol. Des. Educ. 2012 , 23 , 623–636. [ Google Scholar ] [ CrossRef ]
- International Technology Education Association. Standards for Technological Literacy. Content for the Study of Technology (3rd ed.). Available online: https://www.iteea.org/42511.aspx (accessed on 9 June 2022).
- Laal, M.; Ghodsi, S.M. Benefits of collaborative learning. Procedia-Soc. Behav. Sci. 2012 , 31 , 486–490. [ Google Scholar ] [ CrossRef ]
- Wu, Q.; Lu, J.; Yu, M.; Lin, Z.; Zhan, Z. Teaching Design Thinking in a C-STEAM Project: A Case Study of developing the Wooden Arch Bridges’ Intelligent Monitoring system. In Proceedings of the 13th International Conference on E-Education, E-Business, E-Management, and E-Learning (IC4E), Tokyo, Japan, 14–17 January 2022; pp. 280–285. [ Google Scholar ] [ CrossRef ]
- De Bono, E. New Thinking for the New Millennium ; Viking Adult: New York, NY, USA, 1999. [ Google Scholar ]
- Australian Curriculum, Assessment, and Reporting Authority. The Australian Curriculum: Technology. Available online: https://www.australiancurriculum.edu.au/umbraco/Surface/Download/Pdf?subject=Digital%20Technologies&type=F10 (accessed on 9 June 2022).
- Australian Curriculum, Assessment, and Reporting Authority. ACARA STEM Connections Project Report. Available online: https://www.australiancurriculum.edu.au/media/3220/stem-connections-report.pdf (accessed on 9 June 2022).
- Peron, M.; Alfnes, E.; Sgarbossa, F. Learning Through Action: On the Use of Logistics4.0 Lab as Learning Developer. In Proceedings of the 7th European Lean Educator Conference, ELEC 2021, Online, 25–27 October 2021; Powell, D.J., Alfnes, E., Holmemo, M.D., Reke, E., Eds.; Springer: Trondheim, Norway, 2021; Volume 610, pp. 205–212. [ Google Scholar ] [ CrossRef ]
- Zhan, Z.; Zhong, B.; Shi, X.; Si, Q.; Zheng, J. The design and application of IRobotQ3D for simulating robotics experiments in K-12 education. Comput. Appl. Eng. Educ. 2021 , 30 , 532–549. [ Google Scholar ] [ CrossRef ]
- Simonetto, M.; Arena, S.; Peron, M. A methodological framework to integrate motion capture system and virtual reality for assembly system 4.0 workplace design. Saf. Sci. 2021 , 146 , 105561. [ Google Scholar ] [ CrossRef ]
- Carlgren, L.; Rauth, I.; Elmquist, M. Framing Design Thinking: The Concept in Idea and Enactment. Creativity Innov. Manag. 2016 , 25 , 38–57. [ Google Scholar ] [ CrossRef ]
- Buchanan, R. Human Dignity and Human Rights: Thoughts on the Principles of Human-Centered Design. Des. Issues 2001 , 17 , 35–39. [ Google Scholar ] [ CrossRef ]
- Yan, L.; Xiaoying, M.; Jian, L.; Rui, W.; Lihong, M.; Guanxing, X.; Qiuling, G. Cultural Competence: Part I of the 5Cs Framework forTwenty-first Century Key Competences. J. East China Norm. Univ. Educ. Sci. 2020 , 38 , 29–44. [ Google Scholar ] [ CrossRef ]
Click here to enlarge figure
Selection Strategy | Papers Resulting from the Search | Selected |
---|---|---|
Keyword search | 99 | 27 |
First-round snowballing approach | 1721 | 12 |
Second-round snowballing approach | 826 | 3 |
Third-round snowballing approach | 186 | 1 |
Fourth-round snowballing approach | 37 | 0 |
Total | 2869 | 43 |
Design Thinking Competence | Frequency in the Data Set | Codes | Explanation |
---|---|---|---|
Prototype | 32 | Prototype Modelling Build Create Make Fabrication | Creating the original or early solution model, it can be a sketch, or other physical or virtual structure that designed. |
Ideate | 31 | Ideate Design Brainstorming develop possible solutions | Generating alternative ideas that may lead to solutions. |
Define | 18 | Define Understand Problem definition Identify problem Clarify problems and constraints | Actionable problem statement based on insights into the problem situation. |
Test | 15 | Test | Experimenting and gathering feedbacks. |
Explore | 14 | Explore Collect information Data collection Discovery Field Studies Observe | Questioning and collecting information to gain deep understanding of the problem. |
Empathize | 13 | Empathize Human-centeredness Needs-finding Sensitizing Feel | Carrying out design around the needs of users, highlighting human-centred design. |
Evaluate | 9 | Evaluating Appraising | Checking if the design meets the user’s needs. |
Optimize | 8 | Optimization Improve Evolution Iteration Redesign | Refining solution based on user’s feedbacks. |
Dependent Variables | Construct |
---|---|
Subject learning performance | Subject concept |
Subject skill | |
Design thinking | Design thinking concept |
Design thinking process | |
Design thinking work | |
Emotional/social aspect | Attitude |
Interest | |
Satisfaction | |
Desire | |
Acceptance | |
collaboration | |
Skill | Creativity |
Critical thinking | |
Problem solving | |
Productive thinking |
Measurement Instrument | Explanation and Examples |
---|---|
Survey/Questionnaire | Surveys or questionnaires are often used for investigating skills or emotional/social dispositions towards DTIL (e.g., [ , , , , ]). |
Portfolio | Collecting and evaluating students’ design products purposefully and systematically (e.g., [ , , ]). |
Interview | Researcher adopted interview to probe participants’ understanding of DTIL (e.g., [ , , ]). |
Observation | Observation is usually employed to explore participants’ procedural performance in greater detail (e.g., [ , , ]). |
Protocol analysis | Protocol analysis is often adopted to understand the thought process of individual or groups in a natural way, the object of its analysis includes the coded verbal communication, or the thought process being asked to speak out (e.g., [ , , ]). |
Test | To estimate students’ mastery of relevant knowledge, test or examination is usually adopted (e.g., [ , , , ]). |
Design work evaluation | Design work is seen as a direct way to reflect students’ learning outcome, and it is widely implemented in the evaluation of DTIL (e.g., [ , , ]). |
Video recording/Audio recording | In DTIL, researcher recorded the design activities by video or audio so that to understand the participants’ learning process more fully (e.g., [ , , , ]). |
Journal | Journals or diaries from participants is analysed to help understand the process by which learning occurs (e.g., [ , , ]). |
MDPI stays neutral with regard to jurisdictional claims in published maps and institutional affiliations. |
Share and Cite
Li, T.; Zhan, Z. A Systematic Review on Design Thinking Integrated Learning in K-12 Education. Appl. Sci. 2022 , 12 , 8077. https://doi.org/10.3390/app12168077
Li T, Zhan Z. A Systematic Review on Design Thinking Integrated Learning in K-12 Education. Applied Sciences . 2022; 12(16):8077. https://doi.org/10.3390/app12168077
Li, Tingting, and Zehui Zhan. 2022. "A Systematic Review on Design Thinking Integrated Learning in K-12 Education" Applied Sciences 12, no. 16: 8077. https://doi.org/10.3390/app12168077
Article Metrics
Article access statistics, further information, mdpi initiatives, follow mdpi.
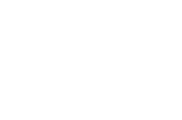
Subscribe to receive issue release notifications and newsletters from MDPI journals
Click through the PLOS taxonomy to find articles in your field.
For more information about PLOS Subject Areas, click here .
Loading metrics
Open Access
Peer-reviewed
Research Article
Design thinking teaching and learning in higher education: Experiences across four universities
Roles Conceptualization, Data curation, Formal analysis, Investigation, Methodology, Project administration, Validation, Writing – original draft, Writing – review & editing
* E-mail: [email protected]
Affiliation Division of Practice Advancement and Clinical Education, Center for Innovative Pharmacy Education and Research, UNC Eshelman School of Pharmacy, University of North Carolina at Chapel Hill, Chapel Hill, NC, United States of America

Roles Conceptualization, Investigation, Methodology, Project administration, Writing – original draft, Writing – review & editing
Affiliations Department of Health Behavior, UNC Gillings School of Global Public Health, University of North Carolina at Chapel Hill, Chapel Hill, NC, United States of America, Office of the Vice Chancellor for Innovation, Entrepreneurship, and Economic Development, University of North Carolina at Chapel Hill, Chapel Hill, NC, United States of America
Roles Conceptualization, Investigation, Methodology, Project administration, Supervision, Writing – original draft, Writing – review & editing
Affiliation Center for Design Thinking, Elon University, Elon, NC, United States of America
Roles Conceptualization, Formal analysis, Writing – review & editing
Affiliation Department of Art, Elon University, Elon, NC, United States of America
Roles Formal analysis, Writing – original draft, Writing – review & editing
Affiliation Office of the Vice Chancellor for Innovation, Entrepreneurship, and Economic Development, University of North Carolina at Chapel Hill, Chapel Hill, NC, United States of America
Roles Conceptualization, Methodology, Project administration, Writing – review & editing
Affiliation Social Science Research Institute and Innovation and Entrepreneurship Initiative, Duke University, Durham, NC, United States of America
Roles Conceptualization, Methodology, Writing – review & editing
Affiliation Department of Graphic Design and Industrial Design, College of Design, North Carolina State University, Raleigh, NC, United States of America
- Jacqueline E. McLaughlin,
- Elizabeth Chen,
- Danielle Lake,
- Wen Guo,
- Emily Rose Skywark,
- Aria Chernik,
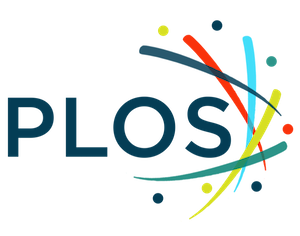
- Published: March 24, 2022
- https://doi.org/10.1371/journal.pone.0265902
- Peer Review
- Reader Comments
A growing body of literature highlights the increasing demand on college graduates to possess the problem finding, problem framing, and problem-solving skills necessary to address complex real-world challenges. Design thinking (DT) is an iterative, human-centered approach to problem solving that synthesizes what is desirable, equitable, technologically feasible, and sustainable. As universities expand efforts to train students with DT mindsets and skills, we must assess faculty and student DT practices and outcomes to better understand DT course experiences. Understanding how DT is taught and experienced within higher education can help schools promote student learning and align their training programs with professional, personal, and civic needs. In this study, surveys were completed by 19 faculty and 196 students from 23 courses at four universities. DT teaching and learning was characterized by three DT practices and five outcomes. Statistically significant differences were found by discipline of study and student type (i.e., graduate vs undergraduate), but not by gender or race/ethnicity. These results can be used to inform the development of classroom-based DT teaching and learning strategies across higher education institutions and disciplines.
Citation: McLaughlin JE, Chen E, Lake D, Guo W, Skywark ER, Chernik A, et al. (2022) Design thinking teaching and learning in higher education: Experiences across four universities. PLoS ONE 17(3): e0265902. https://doi.org/10.1371/journal.pone.0265902
Editor: Alessandro Margherita, University of Salento, ITALY
Received: October 5, 2021; Accepted: March 10, 2022; Published: March 24, 2022
Copyright: © 2022 McLaughlin et al. This is an open access article distributed under the terms of the Creative Commons Attribution License , which permits unrestricted use, distribution, and reproduction in any medium, provided the original author and source are credited.
Data Availability: All data files are available from the Open-ICPSR database ( https://doi.org/10.3886/E151681V1 ).
Funding: The author(s) received no specific funding for this work.
Competing interests: The authors have declared that no competing interests exist.
Introduction
Universities have faced considerable scrutiny in recent years for their apparent failure to adequately equip students with the complex reasoning and problem solving skills thought to be at the core of higher education [ 1 – 3 ]. A growing body of literature highlights the demand on college graduates to simultaneously master the disciplinary knowledge and mindsets necessary to address complex real-world problems [ 4 – 7 ]. These demands, coupled with ongoing concerns about the quality of higher education, have drawn attention to the need to rethink our focus within higher education [ 8 – 11 ].
Design thinking (DT) is an iterative, creative approach to problem finding, problem framing, and problem solving that synthesizes what is desirable to real stakeholders, equitable, technologically feasible and sustainable [ 12 , 13 ]. Most models of design thinking move through (1) inspiration, empathy and problem definition, (2) ideation, (3) prototyping and testing and (4) implementation stages [ 14 ]. By beginning with the goals and needs of stakeholders and engaging in short iteration cycles, DT supports collaborative solutions that roll out with lasting impact [ 12 , 15 ]. Professional design consultancies often use this method to design innovative products or services. Thus, DT is a tool frequently taught in business, engineering and design schools.
A growing number of disciplines are utilizing and teaching DT to solve complex problems, including public health, healthcare, and the liberal arts [ 12 , 16 – 18 ]. The success of innovations developed with the DT process has led to the uptake of DT to solve various challenges, including customer experience and strategic planning, and to support various sectors, including government agencies, non-profits, educational institutions, and community organizations [ 15 ]. Power dynamics can be challenged at the intersection of design for social innovation; this form of participatory design can help students imagine new ways of thinking to solve complex problems [ 19 ]. The human-centered, real-world solutions generated from the DT process have the potential to provide more systemic solutions to difficult social problems, like climate change, poverty, housing instability, and health promotion.
As DT is adopted by a broader audience, there is an onus on educators to equip students across university disciplines with tools and mindsets valuable for addressing these complex, real-world problems. This includes, but is not limited to “situatedness”, self-reflection, empathetic listening, critical observation and creative collaboration [ 20 – 23 ]. DT pedagogy should (1) frame both the situation and the student’s place within the situation; 2) allow for iterative exploration across space and time alongside diverse stakeholders 3) require the generation of divergent possibilities 4) the prototyping and actionable testing of these possibilities and (5) develop sustainable commitments to cultivated change [ 21 ].
Within higher education, we see new centers, programs, and courses being established outside common DT fields (i.e., business, engineering, design) with a focus on teaching DT mindsets and skills, such as Tulane University’s Phyllis M. Taylor Center for Social Innovation, University of Illinois’ Seibel Center for Design, and Design Thinking and Elon University’s Center for Design Thinking. As universities expand efforts to train students with DT mindsets and skills, we must assess faculty and student DT practices and outcomes to better understand DT course experiences. In one single institution study, for example, researchers found that DT requires time and trust which can be constrained by the imposed deadlines of semester-based projects [ 21 ]. In a single course study, students indicated that their “whirlwind” course promoted almost “exponential” growth [ 22 ]. While survey instruments that measure DT practices and outcomes have been validated across diverse workplace settings [ 24 ], the assessment of DT practices and outcomes across higher education is still new.
DT pedagogy in higher education is incompletely understood, particularly in fields beyond traditional design disciplines (i.e., de-disciplined design). We expanded a single institution study of faculty by Lake and Colleagues [ 21 ] in an effort to assess DT teaching and learning (DT-TL) experiences of undergraduate students, graduate students, and faculty at four universities within a southeastern state of the United States. Overall, we set out to answer the following research question: how do faculty and students experience design thinking within higher education courses? To answer this question, we explored the following subquestions:
- What kinds of DT practices are experienced within higher education courses? In what ways do higher education DT practices align with and differ from other industries?
- What kinds of outcomes are experienced within higher education courses? In what ways do outcomes of DT in higher education align with and differ from other industries?
- Is DT a valid construct within higher education teaching and learning?
- What differences exist between groups, such as discipline (business, design & engineering, other), and student type (undergraduate, graduate)?
To answer these research questions, we utilized a mixed methods design that combined faculty and student surveys with semi-structured interviews. This manuscript focuses on the survey data only. Additional qualitative and mixed methods findings are detailed elsewhere [ 25 ].
Survey development
The survey used in this study was adapted from Liedtka and Bahr, who studied DT practices and outcomes among employees of for-profit, non-profit, and government sectors [ 24 ]. Liedtka and Bahr survey items were adapted to align with the context of higher education and additional items were included based on research team experiences. The final survey included 11 items about DT practices, 42 items about outcomes from DT, 11 demographic items (e.g., What is your gender identity ?), and 11 course descriptor items (e.g., Was the term "design thinking" explicitly referenced in the course ?). All DT practices and outcomes items were prompted by the following stem: Please note how often , as a direct result of this specific course , you observed the following [practices/outcomes] and measured on a scale from 1-Never to 5-Almost Always. The research team reviewed the survey for face validity prior to data collection.
Data collection
Students and faculty at four universities in the southeastern United States were recruited for the study during the 2020–2021 academic year. Purposive sampling was used to identify and recruit participants based on their experience with the research focus [ 26 ]. In August 2020, research team members recruited faculty at their home institutions who they knew taught design thinking courses. The email recruitment invited faculty to fill out an initial interest survey about their course, including: course number and title; number of students enrolled in their course; whether their course was open to undergraduate students, graduate students, or both; and number of credit hours associated with the course. The survey also asked faculty for informed consent, contact information, and whether they were interested in participating in an optional semi-structured interview at the end of the semester. Faculty who agreed to participate were also expected to recruit students from their DT course to the study.
Toward the end of the Fall 2020 semester, research team members emailed the faculty survey to consented faculty from their home institutions. Faculty participants were also asked to forward study invitations to students enrolled in their DT courses. The email invitations drafted by our research team included information about the study and a link to the student survey. Students interested in participating in the study provided informed consent in the online survey and were also asked whether they wanted to schedule an optional follow-on interview. Faculty participants received three email reminders regarding study invitations to their students before the end of the Fall 2020 semester.
Data analysis
Survey data were first analyzed using descriptive statistics, with continuous variables reported as mean ± standard deviation (SD) and categorical variables reported as frequency (percent). An exploratory factor analysis was conducted to identify DT teaching and learning (DT-TL) constructs using principal components analysis with varimax rotation and Kaiser rule (ie, eigenvalues < 1.0) for student survey items also included in the Liedka and Bahr study [ 24 ]. Bivariate correlations were calculated using Pearson rho (r p ) and reliabilities were calculated using Cronbach α. Group comparisons were examined using independent t-tests and one-way ANOVA with Bonferroni post hoc analysis. Parametric statistics were considered appropriate due to normality of data and sufficient sample size. Statistical significance was established at α<0.05. All data analysis was performed in SPSS for Windows, v26 (IBM, Armonk, NY).
Ethical considerations, consent
This project was submitted to the UNC Institutional Review Board (#20–2316), Elon University Institutional Review Board (#21–031), Duke Campus Institutional Review Board (#2021–0168), and North Carolina State University (#23502). The submission was approved or determined to be exempt from further review by each review board according to 45 CFR 46.104. Written consent was obtained electronically from all participants at the start of the survey.
Surveys were completed by 19 faculty and 196 students from 23 courses at four universities. The response rate for faculty was 84.2%. Based on the number of students enrolled in each course, our estimated response rate of students was 20.8%. As seen in Table 1 , student participants were predominately white (n = 132, 63.4%), female (n = 126, 64.2%), and majoring in Interdisciplinary Humanities/Social Sciences (n = 105, 53.6%). Eighty-seven percent (n = 170) of students were undergraduate students. Similarly, faculty participants were predominately white (n = 14, 73.7%), female (n = 11, 57.9%), and from Interdisciplinary Humanities/Social Sciences (n = 11, 57.9%).
- PPT PowerPoint slide
- PNG larger image
- TIFF original image
https://doi.org/10.1371/journal.pone.0265902.t001
When asked about the course, most students (n = 149, 76.0%) and faculty (n = 14, 73.7%) indicated that DT was explicitly taught in the course and that they utilized a real-world project (n = 160 (81.6%) students and 13 (68.4%) faculty). On average, students reported spending 69.69% ± 21.65% of their time working in teams. Most students indicated having none or limited DT expertise prior to the course (n = 146, 74.4%) and moderate or extensive expertise after the course (n = 152, 77.5%). Similarly, the number of faculty reporting none (n = 0, 0%) or limited DT expertise (n = 7, 36.8%) prior to the course dropped to 2 (10.5%) after the course .
Table 2 provides item-level responses for DT practices in the current study and two related DT studies using the same survey items. On a five-point scale from 1-Never to 5-Almost Always, student and faculty participants reported that they used all 11 of the DT practices with moderate to high frequency in the course. Faculty indicated that they most commonly followed a structured process (4.16 ± 0.69), created prototypes of ideas (4.11 ± 0.66), and emphasized active listening among team to find shared meaning (4.11 ± 0.88). Similarly, students most commonly emphasized active listening among team to find shared meanting (4.38 ± 0.77), followed a structured process (4.16 ± 0.75), and generated a diverse set of ideas (4.16 ± 0.85). Faculty and students executed real world experiments least frequently (3.16 ± 1.26 and 2.96 ± 1.34, respectively).
https://doi.org/10.1371/journal.pone.0265902.t002
As seen in Table 3 , factor analyses indicated that DT-TL practices can be broadly characterized by three constructs accounting for 59.03% of the variance: Discovery and Ideation (4.04 ± 0.70, 21.67% of variance); Team Formation and Functioning (4.21 ±0.53, 16.02% of variance); and Prototyping and Experimentation (3.44 ±0.94, 21.34% of variance). Survey items loaded into the same three factors as Liedtka and Bahr [ 24 ], with the exception of “Followed a Structured Process,” which loaded to Discovery and Ideation in Liedkta and Bahr (2019) instead of on Team Formation and Functioning in our study. Students who reported that DT was explicitly taught in their course more frequently engaged in Discovery and Ideation than those who indicated DT was not explicitly taught (4.16±0.65 vs 3.42±0.72, p<0.001). Students from Business and Design & Engineering disciplines also more frequently engaged in Discovery and Ideation than those from Interdisciplinary Humanities/Social Sciences (4.21±0.53 vs 3.85±0.69, p = .04; 4.26±0.74 vs 3.85±0.69, p = 0.004 respectively). Undergraduate students reported Prototyping and Experimentation more frequently than graduate students (3.49±0.88 vs 3.07±1.24, p = 0.04). There were no differences in DT practices found by race/ethnicity, or gender.
https://doi.org/10.1371/journal.pone.0265902.t003
Table 4 provides factors loadings for outcomes of DT in higher education courses, which are broadly characterized by five constructs accounting for 63.00% of the variance: Implementation Support (4.00 ± 0.70, 18.51%), Psychological Benefits and Motivation (4.15 ± 0.64, 14.13%), Relationships and Trust (3.76 ±0.82, 12.74%), Quality of Solutions Generated (4.21 ± 1.75, 8.98%), and Individual Adaptation and Flexibility (4.13 ± 0.66, 8.64%). Students who reported that DT was explicitly taught more frequently experienced Psychological Benefits and Motivation than those who indicated DT was not explicitly taught in their course (4.21±0.62 vs 3.85±0.87, p = 0.008). There were no differences in outcomes of DT found by race/ethnicity, gender, or student type (ie, undergraduate vs graduate).
https://doi.org/10.1371/journal.pone.0265902.t004
As seen in Table 5 , four of the five outcomes factors contained similar items and items that differed. Factor 3, for example, emphasized Relationships in both studies and included the survey item Built new relationships locally that continued after the initial project was completed . However, in the current study, other items in that factor addressed Trust (e.g., Built trust among team members) while other items in the Liedtka and Bahr study addressed Resources (e.g., Expanded access to new resources for individuals and teams .)
https://doi.org/10.1371/journal.pone.0265902.t005
All correlations between the three DT practice and five outcome constructs were statistically significant, ranging from positive, moderate relationships (r p = 0.33) to positive, very strong relationships (r p = 0.76) ( Table 6 ). Cronbach alpha exceeded .6 for seven of the eight DT constructs (0.53 ≤ α ≤ 0.89), suggesting that the items used to create each construct demonstrated acceptable internal consistency.
https://doi.org/10.1371/journal.pone.0265902.t006
This study explored faculty and student experiences with DT in courses from various disciplines within four universities. Given the increasing uptake of DT in higher education and across professional fields, this study is timely and critical for understanding the types of DT practices and outcomes experienced, and ways in which DT in these settings might vary among different industries and stakeholders. This work extends research conducted in workplace settings [ 24 ] and compliments studies exploring conceptual frameworks and uses of DT in education [ 27 , 28 ]. Luka, for example, integrated multiple DT models to design an international English course that engaged learners in a four-phased learning cycle of experiencing, reflecting, thinking and acting [ 27 ] while Wrigley and Straker described the Educational Design Ladder, which illustrates the organization of a multidisciplinary DT program [ 28 ].
DT-TL constructs
The results of this study suggest that DT-TL is a valid construct in its own right within the context of higher education. Specifically, the factor analysis revealed 8 distinct factors with high factor loads and the majority of variance accounted for by the analysis, providing support for content specificity. While the 3 DT-TL practice constructs and 5 outcomes constructs are aligned with those described in other contexts [ 24 ], they also embody items differently, which may be attributable to the different processes and contexts associated with student learning in higher education environments. As noted below, the experiences of students and faculty in DT-TL–as indicated by item ratings and construct scores–are likely influenced by interactions with others, constraints of higher education systems (e.g., semester timelines), and disciplinary differences in DT-TL approaches (e.g., business vs humanities). Additional research is needed to understand how and why DT is experienced differently by various stakeholders, and which organizational aspects of these contexts might mediate or support relevant outcomes.
DT-TL practices
Although participants indicated frequently utilizing all DT practices, some were used more than others. Namely, participants indicated engaging in Team Formation and Functioning most frequently, which aligns with previous studies [ 21 , 24 ] and likely reflects DT’s commitment to collaborative project-based problem-solving. In contrast, participants engaged in Protyping and Experimentation least frequently, which also aligns with previous studies in higher education [ 21 ]. We speculate that a lack of prototyping and experimentation could be a byproduct of dominant approaches to classroom learning that deemphasize the need for experiential and experimental practices and the constraints of a semester-long course (i.e., limited time available to iterate through the full DT process). While there are many student benefits to experiential learning [ 29 ], applied learning [ 30 ], process-based learning [ 31 ], and service-learning [ 32 ], they are time-consuming and resource intensive to execute and come with their own limitations and challenges [ 32 ]. We recommend faculty consider generating lower-stakes, quicker-paced student learning opportunities to prototype and test in addition to offering consecutive semester-long courses to ensure students are provided opportunities to develop these skills.
Compared to research in workplace settings [ 24 ], our faculty and students reported a greater frequency of the following front-end practices: followed a structured process , emphasized active listening among team to find shared meaning , and focused problem definition on the user’s perspective rather than the organization’s . On the other hand, some practices associated with the later phase of the DT process were reported at lower frequency by faculty and students in our study compared to participants in the Liedtka and Bahr [ 24 ] study, including: moved multiple ideas into prototyping , got feedback form users and other stakeholders on prototype , and executed real world experiments .
Outcomes of DT-TL
Recent research suggests that DT can empower students to design desirable, feasible, transdisciplinary solutions that promote practical and sustainable outcomes [ 22 , 33 ]. Our results align with Lake and colleagues [ 22 ], who also found that the Quality of Solutions Generated was the most frequently experienced outcome, highlighting the process of engaging students in the DT process as a means for solving complex problems. Not surprisingly, Quality as an outcome had a moderately strong, positive relationship with the practice of Team Formation and Functioning , highlighting the potential benefits of well-designed, high-structured teamwork within courses.
In addition, literature highlights potential cognitive and behavioral benefits of DT, showing positive cognitive and behavioral changes for learning and decision-making [ 28 , 34 ]. Our results align with this literature, suggesting that participants frequently experienced Psychological Benefits and Motivation (e.g., kept people motivated to work on a project to achieve impact ). These findings are crucial for educators seeking evidence that DT teaching practices provide students with skills and mindsets for more inclusively and resiliently addressing complex, real world challenges. Both benefits highlight the importance of establishing relevance between student learning and real-world situations through DT pedagogies.
We posit that our outcomes of DT-TL were similar and different from those of Liedtka and Bahr [ 24 ] due to our different study populations and contexts. We adapted the survey instrument for faculty and students in higher education institutions involved in semester-long classes whereas Liedtka and Bahr [ 24 ] designed it for employees of for-profit, non-profit, and government entities. In our sample, the items on trust ( built trust among team members , built trust between problem-solving teams and other stakeholders ) loaded alongside other relationship items whereas, in Liedtka and Bahr [ 24 ], those items loaded onto its own factor. In academic settings, we often talk about relationship-building and trust together, especially as we discuss community engagement, so this makes sense. In community-based participatory research in particular, relationship-building and trust-building go hand-in-hand [ 35 , 36 ].
We also found that survey items kept people motivated to work on a project to achieve impact , increased a sense of ownership and acceptance of a solution , and increased appreciation for use of data to help drive decisions loaded with the factor on psychological benefits rather than the improved implementation and adaptation factor from Liedtka and Bahr [ 24 ]. These three items mark shifts in individual mindsets so this grouping makes sense. Relatedly, the new factor we proposed is termed “Individual Adaptation and Flexibility” and centers around growth mindset and a willingness to learn and change.
DT-TL group differences
Our findings generally indicate that DT-TL practices and valued outcomes are prevalent across disciplines, providing early insights into the potential merits of DT-TL as an interdisciplinary process that is valued across institutions. Several group comparisons warrant discussion and further research, such as undergraduate students experiencing Prototyping and Experimentation more frequently than graduate students. Although graduate degree programs typically immerse graduate students in research, we posit that the structure and processes within graduate programs–which tend to include close oversight from an expert faculty member in a focused area of study–may limit the creativity and brainstorming opportunities afforded to undergraduates. Alternatively, if graduate students experience Prototyping and Experimentation frequently in their degree program, they may perceive this practice as less frequent in DT coursework compared to undergraduate students who are not engaged in similar research programs. As it relates to race and ethnicity, lack of differences should be interpreted with caution since data were collected from predominantly white institutions and the sample sizes for subgroups were relatively small. However, this finding should be explored further given legitimate concerns that DT practices can further privilege those with privileged identities [ 37 , 38 ].
Future directions
This study marks an important step in understanding DT across institutions and disciplines within higher education and across educational and professional divides. Our findings, coupled with those from K-12 education–where DT is lauded as an integral cognitive process involving creation, collaborative sense-making, reasoning with evidence, experimentation, and evaluation [ 39 – 41 ]–pose several intriguing opportunities for next steps.
Taken together, K-12, higher education, postgraduate, and professional studies contribute to the growing body of research that demonstrates clear DT benefits for students throughout their educational careers and beyond. As education faces ongoing scrutiny about its relevance and value, educators must adopt strategies that enable students to address increasingly complex real-world challenges. In Doctors as Makers , for example, Baruch implores medical curricula to foster creative and critical thinkers that can work through not-knowing, seek compassionate solutions, and explore questions differently within an environment supportive of iterative development [ 42 ]. Understanding the ways in which DT compliments traditional problem-solving frameworks, such as the scientific method and clinical decision making, could be an important step toward optimizing how DT-TL is integrated into our curricula [ 16 ].
With its emphasis on situated and relational problem-solving frameworks, DT-TL also aligns with “problem-posing” pedagogical theory and praxis that is core to various academic disciplines, such as innovation and entrepreneurship. In Pedagogy of the Oppressed , Freire described problem-posing education as a shift from a content-centric, hierarchical educational model to a contextually responsive, co-creative one [ 43 ]. Specifically, DT-TL maps seamlessly onto many of the problem-posing processes taught in innovation and entrepreneurship education, particularly to the discovery process (e.g., problem identification through understanding stakeholder needs ) and the development process (e.g., iterative testing and evaluation of prototypes ). Similarly, other pedagogical models, such as community-based, project-based, and problem-based-learning include components that align closely with DT. Since institutions who make DT a central strategic focus are more likely to bolster and sustain a competitive advantage [ 44 ], more research is needed in higher education to understand the potential prevalence and relationship of DT-TL to pedagogical praxis and other pedagogical models (e.g., problem-based learning) across various disciplines.
Moving forward, we encourage DT-TL educators to extend our work by collecting similar data, developing improved quantitative measures, and implementing longitudinal studies with faculty, students, and project partners. Larger, more representative samples will promote generalizability and enable analyses and sub-analyses examining additional institution- (e.g., university size, Carnegie Classification, institutional control) and faculty-level (e.g., number of years teaching DT, discipline of home department or school, rank or track) factors. Moreover, it would be helpful to know how often students experience DT prior to taking these courses, shortly after taking these courses, and several months or years after taking the courses. This could allow us to better establish causality and attribute changes to the specific courses. Additional research should also be conducted to understand DT-TL outcomes for co-curricular opportunities, such as student organizations and internships to determine what other opportunities in higher education settings can yield similar or better outcomes.
Limitations
With regards to study limitations, we encountered standard challenges with response rates, missing data, and non-response. We recruited faculty and students from four predominantly White higher education institutions in the same state and had a small sample size of faculty at each institution. Data also reflect student and faculty perception at the end of a course–not the long-term outcomes/value of these practices for them or for their project collaborators. That being said, our results are not meant to be generalizable to all DT courses in across higher education settings. Rather, they provide an important first glimpse into DT-TL practices across multiple universities and disciplines.
College students must be equipped with the critical, creative, and collaborative skills needed to address society’s increasingly complex and difficult challenges. As this study further verified, DT helps to generate trust across collaborators, fosters the motivation needed to sustain problem-solving efforts, and increases the quality of solutions generated. This study demonstrated the validity of DT across disciplines and universities, the ways in which DT practices and outcomes are experienced in higher education, and a number of important differences between DT practices within higher education and other sectors. Extending this work to include additional universities and research methodologies is critical for providing insight into enhancing the value of DT pedagogies.
Supporting information
S1 table. outcomes of design thinking coursework..
https://doi.org/10.1371/journal.pone.0265902.s001
Acknowledgments
The authors would like to acknowledge the faculty and students who participated in the study.
- 1. Arum R, Roksa J. Academically adrift: Limited learning on college campuses. Chicago: University of Chicago Press; 2011.
- View Article
- Google Scholar
- 4. Bourn D. Understanding global skills for 21st century professions. 1 st ed. London: Palgrave Macmillan; 2018.
- 6. Rainie L, Anderson J. The future of jobs and jobs training. Washington, D.C.: Pew Research Center; 2017.
- 8. Boud D, Falchikov N. Rethinking assessment in higher education: Learning for the longer term. 1 st ed. London: Routledge; 2007. https://doi.org/10.1016/j.nedt.2006.04.007 pmid:16815600
- 9. Fallis G. Rethinking higher education: Participation, research, and differentiation. 1 st ed. Montreal: McGill-Queen’s Press-MQUP; 2013.
- 10. Taylor MC. Crisis on campus: A bold plan for reforming our colleges and universities. 1 st ed. New York: Knopf; 2010.
- 11. Wagner T. The global achievement gap: Why even our best schools don’t teach the new survival skills our children need-and what we can do about it. 1 st ed. New York: Basic Books; 2010.
- PubMed/NCBI
- 13. IDEO. The field guide to human-centered design: Design kit. San Francisco, CA: IDEO; 2015.
- 14. Hoffman L. 10 Models for Design Thinking. 2016 [cited 2021 Aug 10]. p. 674. Available from: https://libhof.medium.com/10-models-for-design-thinking-f6943e4ee068
- 19. Lee R. An Examination of Participatory Design Framework in a Class Project in Higher Education. 1 st ed. Toronto: University of Toronto; 2018.
- 23. Royalty A, Oishi LN, Roth B. Acting with creative confidence: Developing a creative agency assessment tool. In: Plattner H, Meinel C, Leifer L, editors. Design thinking research. New York: Springer; 2014. pp. 79–96.
- 24. Liedtka J, Bahr KJ. Assessing design thinking’s impact: Report on the development of a new instrument. Darden Working Paper Series; 2019.
- 25. Guo W, Lake D, Cox M, Chen E, McLaughlin JE. De-disciplining and democratizing design thinking pedagogies in higher education. Teach Learn Inq; 2022. Forthcoming.
- 26. Schutt J. Investigating the social world: The process and practice of research. 5th ed. Thousand Oaks: Sage; 2006.
- 37. Costanza-Chock S. Design justice: Community-led practices to build the worlds we need. 1 st ed. Cambridge; MIT Press: 2020.
- 43. Freire P. Pedagogy of the oppressed. 4ed. New York: Continuum. 2018.

Design Thinking in Education: Perspectives, Opportunities and Challenges
Chat with Paper
Fostering Teamwork through Design Thinking: Evidence from a Multi-Actor Perspective
Using design thinking method in academic advising: a case study in a college of pharmacy in saudi arabia, design thinking traits and cognitive passive resistance: mediating effect of linear thinking, this school is made for students: students’ perspectives on pbl, creating effective physical learning spaces in the digital age – results of a student-centered design thinking workshop, a multi‐dimensional framework of organizational innovation: a systematic review of the literature, design thinking: a fruitful concept for it development, design thinking pedagogy: the educational design ladder, activity theory in hci: fundamentals and reflections, using concept maps in qualitative research.
documentary
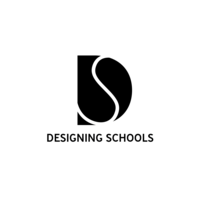
What is Design Thinking in Education?
In a world where artificial intelligence, exemplified by tools like ChatGPT, is reshaping our world, the human touch of design thinking becomes even more crucial. You might already be familiar with design thinking and curious about how to harness it alongside AI, or perhaps you’re new to this method. Regardless of your experience level, I’m going to share why design thinking is your human advantage in an AI-world. We’ll explore its impact on students and educators, particularly when integrated into the curriculum to design learning experiences that are both innovative and empathetic.
Back in 2017, I spearheaded a two-year research study at Design39 Campus in San Diego, CA, focusing on how educators used design thinking to transcend traditional educational practices. This study was pivotal in understanding how to scale from pockets of innovation to a culture of innovation. It’s rare to see a public school integrate these practices, and I always wondered, “Why is this the exception and not the norm?” How might design thinking when combined with AI tools, complement standards-based curricula by prompting students to tackle real-world challenges. We investigated the methods educators used to learn about design thinking and how they crafted learning experiences at the nexus of knowledge, skills, and mindsets, aiming to foster creative problem-solving in an increasingly AI-integrated world. The results revealed it had nothing to do with the technology. It had to do with people.
Design thinking is both a method and a mindset.
What makes design thinking unique in comparison to other frameworks such as project based learning, is that in addition to skills there is an emphasis on developing mindsets such as empathy, creative confidence, learning from failure and optimism.
Seeing their students and themselves enhance and develop their skills and mindset of a design thinker demonstrated the value in using design thinking and fueled their motivation to continue. In addition, it strengthened their self-efficacy and helped them embrace, not fear change.
The results indicate strong agreement amongst the educators between developing in demand skills such as creativity, problem finding, collaboration and communication and practicing design thinking.
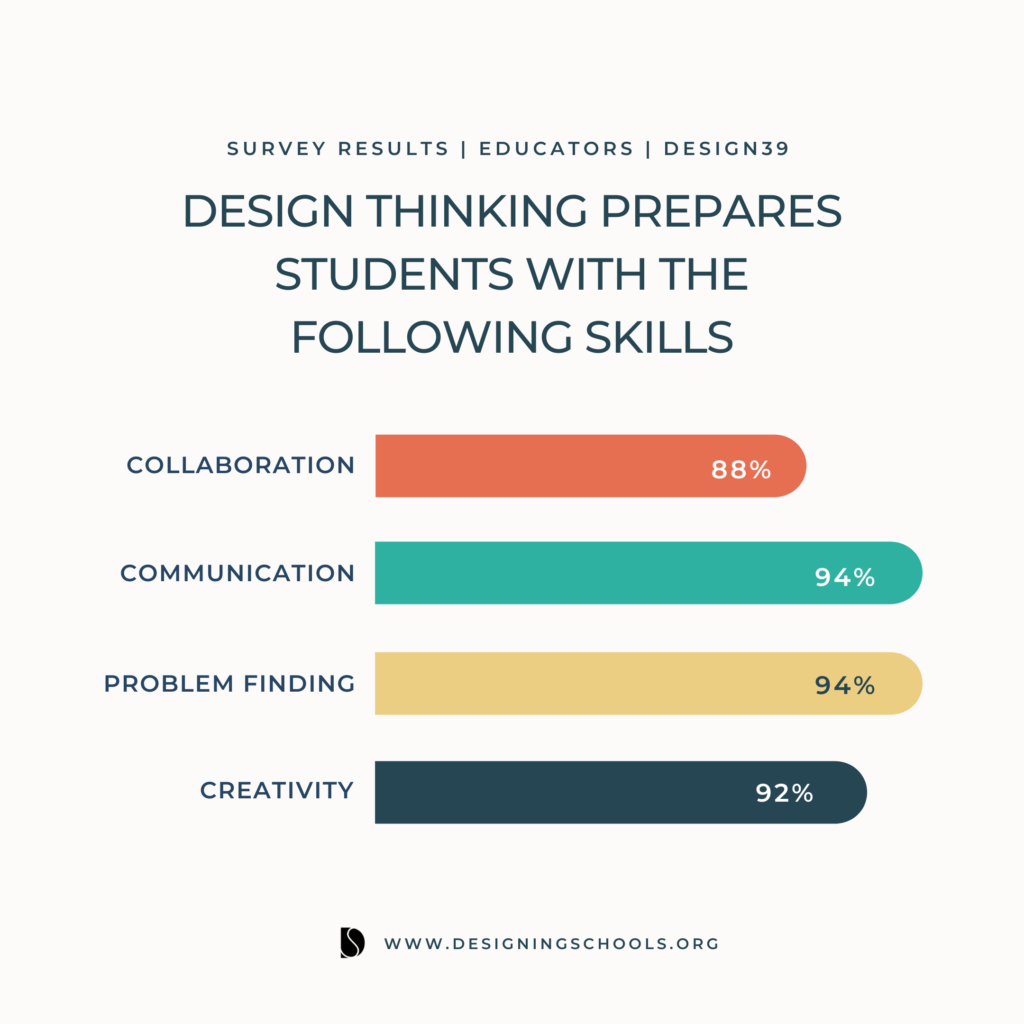
As workplaces determine how to leverage new and emerging technologies in ways that serve humanity, the two critical skills expected will be the ability to solve unstructured problems and to engage in complex communication, two areas that allow workers to augment what machines can do (Levy & Murnane, 2013.)
Brynjolfsson and McAfee (2014) call this era, “The Second Machine Age,” characterized by advances in technology, such as the rise of big data, mobility, artificial intelligence, robotics and the internet of things. The World Economic Forum calls this era, “The Fourth Industrial Revolution.”
Regardless of the name we give this era, Schwab warned, as did Brynjolfsson and McAfee, that failure by organizations to prepare and adapt could cause inequality and fragment societies.
That era that we once talked about, is not here.
The rise of generative Ai.
As Erik Brynjolfsson shares, “There is no economic law that says as technology advances, so does equal opportunity.” The World Economic Forum reinforces this by sharing, that while the dynamics of today’s world have the potential to create enormous prosperity, the challenge to societies, particularly businesses, governments and education systems, will be to create access to opportunities that will allow everyone to share in the prosperity.
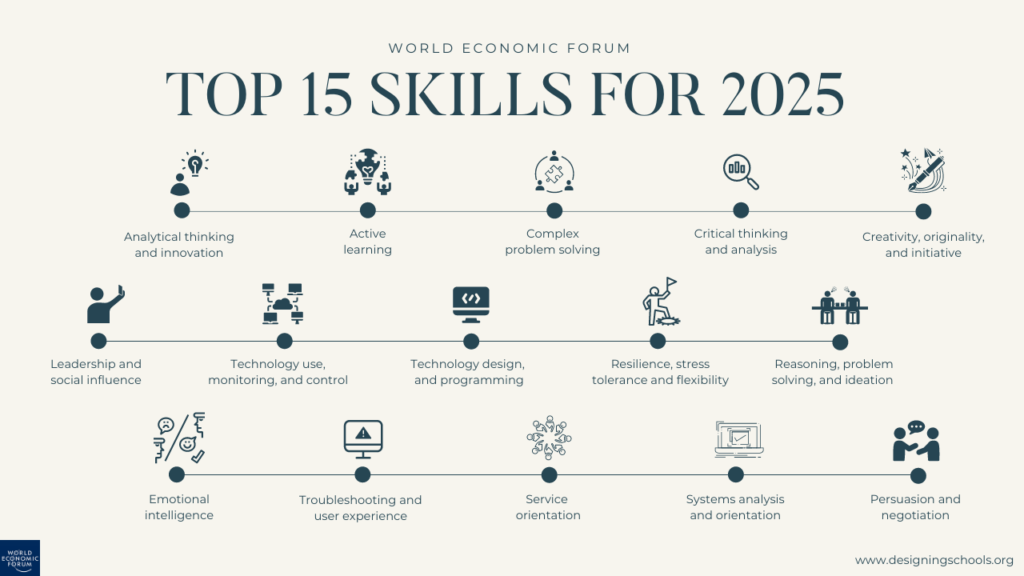
Schwab, Brynjolfsson and McAfee advocate for schools being able to play a powerful role in shaping a future that is technology-driven and human-centered. Design thinking, a human-centered framework is one method that can provide educators with the skills and mindset to navigate away from the traditional model established during the industrial area. To a learner-centered vision where we design learning experiences at the intersection of knowledge, skills, and mindsets.
The Future of Work
Designing schools for today’s learner is not just about solving a workforce or technology challenge. It’s also about solving a human challenge, where every individual has the access and opportunity to reach their potential.
Despite the changing expectations of the workplace brought forth by this era, today’s education systems largely remain unchanged. Leaving graduates without the knowledge, skills and mindsets to thrive in future workplaces and as citizens. Furthermore, the lack of equity has led to what Paul Attewell calls a growing digital use divide deepening the fragmentation of society.
A decade ago, some of the most in-demand occupations or specialties today did not exist across many industries and countries. Furthermore, 60% of children in kindergarten will live in a world where the possible opportunities do not yet exist (World Economic Forum, 2017).
In Technology, Jobs and the Future of Work, McKinsey states that 60% of all occupations have at least 30% of activities that can be automated. 40% of employers say lack of skills is the main reason for entry level job vacancies. And 60% of new graduates said they were not prepared for the world of work in a knowledge economy, noting gaps in technical and soft skills. Before our experience with ChatGPT I’m reminded of Imaginable by Jane McGonigal where she shares, “Almost everything important that’s ever happened, was unimaginable shortly before it happened.”
With an influx of technology over the past decade, with iPads and Chromebooks, and now the acceleration of AI technology, particularly over the past year, we have to wonder what gaps exist that prevent us from accelerating and scaling the change we want to see in schools.
One reason is that this challenge is complex and overwhelming. This is where design thinking practices are helpful in moving from idea to impact. Design thinking practices provide the structure and scaffolds needed to take a complex idea and simplify it.
The Design Thinking Process
Too often design thinking is seen as a series of hexagons to jump through. Check off one and move onto the next. Design thinking is a non-linear framework that nurtures your mindset toward navigating change.
It can be used in three areas:
- Problem finding
- Problem solving
- Opportunity exploration
The design thinking model is nonlinear. Resulting in a back and forth between the stages of inspiration, ideation and implementation, in an effort to continuously improve upon their potential solution (Shively et al., 2018). These stages were expanded by the d.School into empathy, define, ideate, prototype and iterate. In fact, there are many exercises that can be used to apply each area of the process.
Let’s walk through each phase. Then I’ll share examples of how it is being used. I also want to preface this by saying that simply going through these stages is where most people misunderstand design thinking and don’t see the results they hoped for. These phases are here to help you develop an action-oriented mindset. Moving from identifying a problem to designing and then testing a solution to quickly get feedback. Each of these phases have numerous exercises to also help facilitate experiences based on your scenario.
Phase 1: Empathy
When you begin with empathy, what you think is challenged by what you learn. This alone is what makes design thinking so unique and is the first phase. During the empathy stage, you observe, engage and immerse yourself in the experience of those you are designing for. Continuously asking, “why” to understand why things are the way they are.
This phase is where we see the most challenges, yet this phase is the most critical. An empathy map is probably the most common exercise. Yet there are others such as, “Heard, Seen, Respected.” Another challenge in this area is not speaking directly to the user. For example, I’ve sat in many “design thinking” experiences where the group will speculate on behalf of the users. For example, educators speculating about parents, administrators speculating about teachers.
The purpose behind an empathy exercise is that when we begin with empathy, what we think is challenged by what we learn. While you can practice with each other, ultimately you must speak directly to who you are designing for.
Phase 2: Define
During the define stage you unpack the empathy findings and create an actionable problem statement often starting with, “how might we…” This statement not only emphasizes an optimistic outlook, it invites the designer to think about how this can be a collaborative approach.
Phase 3: Ideate
During the ideate phase you generate a series of possibilities for design. The focus here is quantity not quality. As you want to generate as many possibilities to see how they may merge together. As Guy Kawasaki shares, “Don’t worry be crappy.” Feasibility is not important at this step. Rather the key is to not think about what is possible but what can be possible. At the end, one of the ideas, or the merging of many ideas, is chosen to expand upon in the next phase.
This is another phase where we see challenges. It is not enough to simply tell someone to get a piece of paper and then come up with lots of ideas. As adults, this is incredibly challenging and is also a muscle that needs to be developed. In fact, one of my favorite exercises is 1-2-4-all. Another is walking questions, where the prompt begins with “What if…” and then after each person writes something it is handed to the person on their right.
Phase 4: Prototype
During the prototype phase, ideas that were narrowed down from ideation are created in a tangible form so that they can be tested. During this phase, the designer has an opportunity to test their prototype and gain feedback.
Phase 5: Iteration
By quickly testing the prototype, the user can refine the idea. And have a deeper understanding to go back and ask questions to the group they are designing for. The feedback received from the user allows the designer to engage in a deeper level of empathy to refine the questions asked and the problem being defined. This brings us back to phase 1.
You can find more of these exercises to lead your group through each phase at sessionlab.com .
As schools strive to create student learning experiences that prepare them for their future, design thinking can play a critical role in complementing students’ knowledge with the skills and mindsets to be creative problem solvers.
Examples of Design Thinking in K12
While new approaches tend to be viewed with skepticism, an increasing number of studies are coming forward reflecting the promise of transferability of skills and mindsets from the classroom to real-world problems when utilizing design thinking. As expectations are raised about what student skills and mindsets are needed, the level of support for educators must increase as well to experience success in new strategies and the outcomes they promise.
When student learning experiences include design thinking, their skills continue to be enhanced and developed. This in turn allows them to apply these strategies to be problem finders and problem solvers. Helping them be more comfortable with change and empowering them to solve unstructured problems. And work with new information, gaining knowledge, skills and mindsets that cannot be found in the confines of a textbook.
In “The Second Machine Age,” the authors share:
Technological progress is going to leave behind some people, perhaps even a lot of people, as it races ahead. As we’ll demonstrate, there’s never been a better time to be a worker with special skills or the right education. Because these people can use technology to create and capture value. However, there’s never been a worse time to be a worker with only “ordinary” skills and abilities to offer, because computers, robots, and other digital technologies are acquiring these skills and abilities at an extraordinary rate. The Second Machine Age | Erik Brynjolfsson | Andrew McAffee
Design thinking strengthens the mindsets and skills that today’s world demands with the ability to become creative problem solvers. Through nurturing the skills and mindsets developed through engaging in design thinking, schools can create more equitable use environments for all learners that leverage technology to accelerate creative tasks that can bridge the digital use divide.
Case Study 1: Design Thinking in Grade 6
A recent study by the Stanford Graduate School of Education highlights that through instruction, students transfer design thinking strategies beyond the classroom. And that the biggest benefits were to low-achieving students (Chin et al., 2019).
The study included 200 students from grade 6. The researchers worked with the educators during class time to coach half the group of students on two specific design thinking strategies. And then assigned them a project where they could apply these skills.
The two strategies included seeking out constructive feedback and identifying multiple possible outcomes to a challenge. Each of these strategies were designed to prevent what the researchers called, “early closure”. Identifying the potential solution before examining the problem.
After class the students were presented with different challenges to see how they would approach them. The students who were taught about constructive criticism asked for feedback when presented with the new challenge and were more likely to go back and revise their work.
This area was significant, as a pre-test revealed that low-achieving students were behind their high achieving peers when seeking out feedback, a gap that the researchers say disappeared after classroom instruction, highlighting the need for this to be taught to all students, not just advanced students in electives.
As Attewell shares, “Placing computers in the hands of every student is not a solution because the challenge lies in addressing the “ digital use divide – changing the tasks that students do when provided with computers.”
He further highlights the students who gain the types of skills highlighted by the Future of Jobs Report are white and affluent students. These students are more likely to use technology to develop trending skills with greater levels of adult support. Whereas minority students are more likely to use it for rote learning tasks, with lower levels of adult support.
While design thinking is often found in pockets, presented to students already interested in this area, or the students who are in certain electives, the study led by the Stanford Graduate School of Education demonstrates the advances that can be made when this is offered to all students.
Case Study 2: Design Thinking in Geography
Another study (Caroll et al., 2010) focused on the implementation of a design curriculum during a middle school geography class. And explored how students expressed their understanding of design thinking in classroom activities, how affective elements impacted design thinking in the classroom environment and how design thinking is connected to academic standards and content in the classroom. The students were a diverse group with 60% Latino, 30% African-American, 9% Pacific Islander and 1% White.
The task was for students to use the design process to learn about systems in geography. The study found that students increased their levels of creative confidence. And that design thinking fostered the ability to imagine without boundaries and constraints. A key element to success was that educators needed to see the value of design thinking. And it must be integrated into academic content.
A challenge often associated with design thinking in education is not integrating it into mainstream education as an equitable experience for all learners despite showing that lower achieving students benefit more (Chin et al, 2019).
If students are to experience dynamic learning experiences, then organizations must raise the level of support for educators and give them the time and space to learn and integrate design thinking.
How Educators Use Design Thinking
Educators are facing a number of challenges in their professional practice. Many of the requirements today are tools and methods they did not grow up with. Furthermore, the profession is tasked with designing new methods often within traditional systems that have constraints that may serve as roadblocks to change (Robinson & Aronica, 2016).
A 2018 study by PwC with the Business Higher Education Forum shared that an average of 10% of K-12 teachers feel confident incorporating higher-level technology that affords students the opportunity to use technology to design learning that is active, not passive.
As a result, students do not spend much time in school actively practicing the higher-level trending skills expected by employers. Moreover, the report shows that more than 60% of classroom technology use is passive, while only 32% is active use. While the study suggests that many teachers do not have the skills to engage students in the active use of technology, 79% said they would like to have more professional development for how to leverage technology to design learning that is active.
Case Study 3: Design39 Campus
As I shared earlier I led a two-year research study at Design39 Campus. The study examines how it helped teachers evolve their practice. At Design39 teachers are called “Learning Experience Designers” (LEDs). Borko and Putnam (1995) share that how educators think is related to their knowledge. To understand how LEDs are using design thinking to complement the standards-based curriculum, it was important to understand how they acquired and applied this knowledge.
Despite design thinking having its roots outside of education, when asked, “What does design thinking mean to you?” The LEDs identified many commonalities amongst their own work as educators and design thinking. Moreover, they appreciated the alignment of their work with the vocabulary and structure of the design thinking framework.
Over 50% of the LEDs interviewed identified design thinking as providing them with a common vocabulary and structure for what they already do. The LEDs identified educators as inherent design thinkers due to the shared human-centered focus of working with users. In this experience educators design challenges with cyclical learning tasks involving testing, feedback and iteration, and a design mindset to address the wide variety of complex problems within their individual classrooms and across education organizations.
One LED shared:
I just look at it as a process, a process in my mind that we kind of naturally go through as educators, and so with the design thinking process I feel that it is codifying what we do and so we start off always in empathy and empathy is the heart of design thinking and so we are problem solving, who are we problem solving for – people, our learners and so this entire process that we go through of brain dumping it, trying it, getting feedback and coming back to it again so that we can make sure we were really insightful about what the problem really was for the users and we continue around this process to fine tune a potential solution is the design thinking process. Learning Experience Designer | Design39 Campus
One of the ways mastery of knowledge is demonstrated is by teaching others. To assess their mastery of design thinking in education, learning experience designers were asked to describe their confidence in teaching someone else how to integrate design thinking into their curriculum.
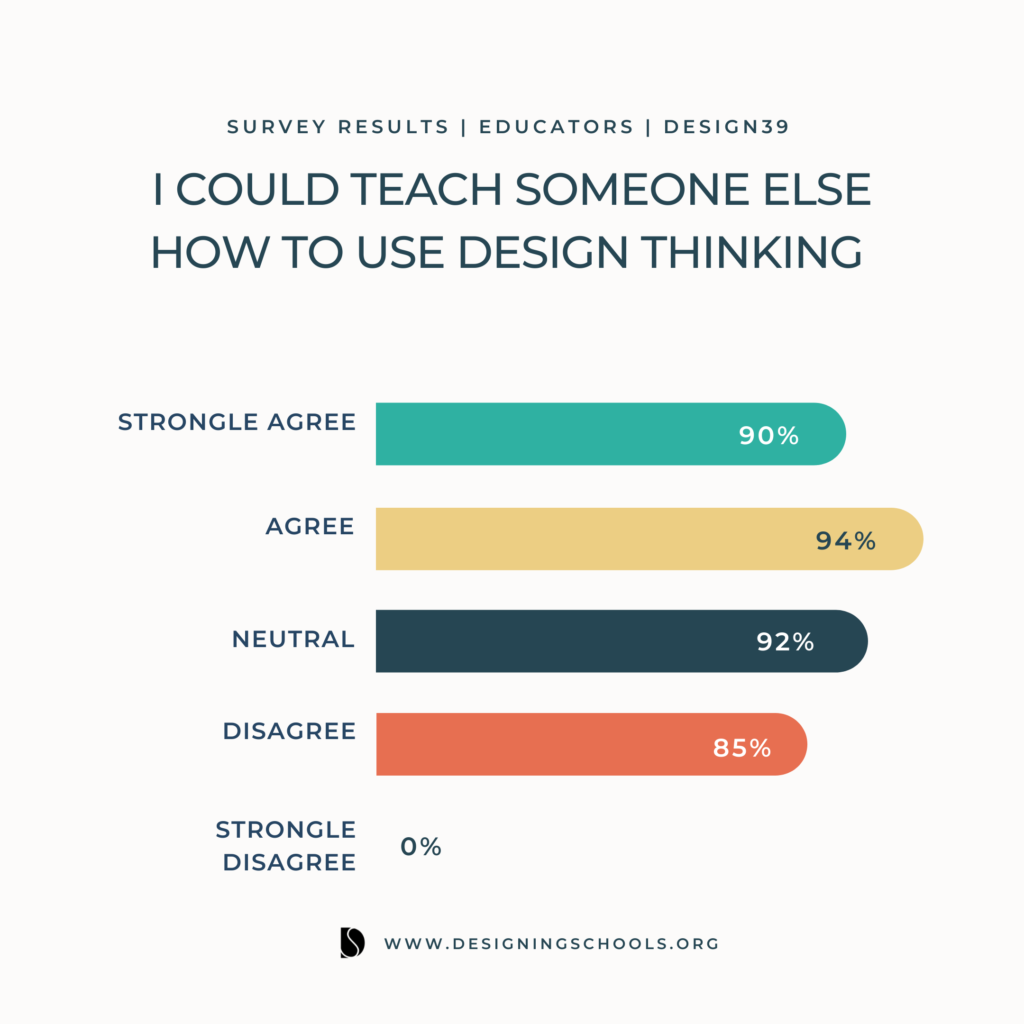
Many LEDs acknowledged that although this is what it often looked like in the first year of the school opening, they have since had the time, space and collaborative opportunities to explore and create deeper integration. This was a point of reference mentioned by 78% of LEDs.
I think a lot of people see design thinking as one science activity, we design think everything from rules to problems that come up in the playground, it’s all through the day, they (the learners) are always looking for problems to solve. Learning Experience Designer | Design39 Campus
In another example, four LEDs made a note using the exact same language that “design thinking is not always cardboard and duct tape.” What allows them to design learning that is more meaningful one LED highlighted:
Not every day is about using duct tape and cardboard, sometimes to do the design to solve the problems you have to hunker down and read and research and so some days, design thinking is highlighting and taking notes. Learning Experience Designer | Design39 Campus
Another LED elaborated on this idea by sharing that
Design thinking is a way of thinking, not always a product that is created at the end. Learning Experience Designer | Design39 Campus
LEDs in all focus groups shared how ultimately design thinking was an opportunity to design lessons that are “ bigger than we are .”
This allowed for the LEDs to design learning experiences. With this, the end result was not to just design a potential solution to a challenge that was identified. Or to simply go from one standard to another, checking off boxes along the way, but that the solution, the work the learners were doing lived beyond the classroom for an authentic audience, where learners are working on real world problems and presenting their solutions to a real world audience.
Almost all of the LEDs shared that to them design thinking was a mindset. It is a process of inquiry that allowed for a more human centered environment where the learner was the focus.
This highlighted a critical shift in the culture at Design39, an element Sarason (2004) discussed in saying no one ever asks:
“Why is school not a place where educators learn as well?”
Bring a Design Thinking Workshop to Your School
We’ve invested in technology. Now it’s time to invest in people. Let’s discuss how design thinking practices can enhance the work you are doing in your school, giving everyone the mindset and skills to navigate change with enthusiasm and optimism. Use this calendar to schedule a time with Sabba to discuss bringing a workshop to your school. Workshops can be delivered both virtually and in-person.
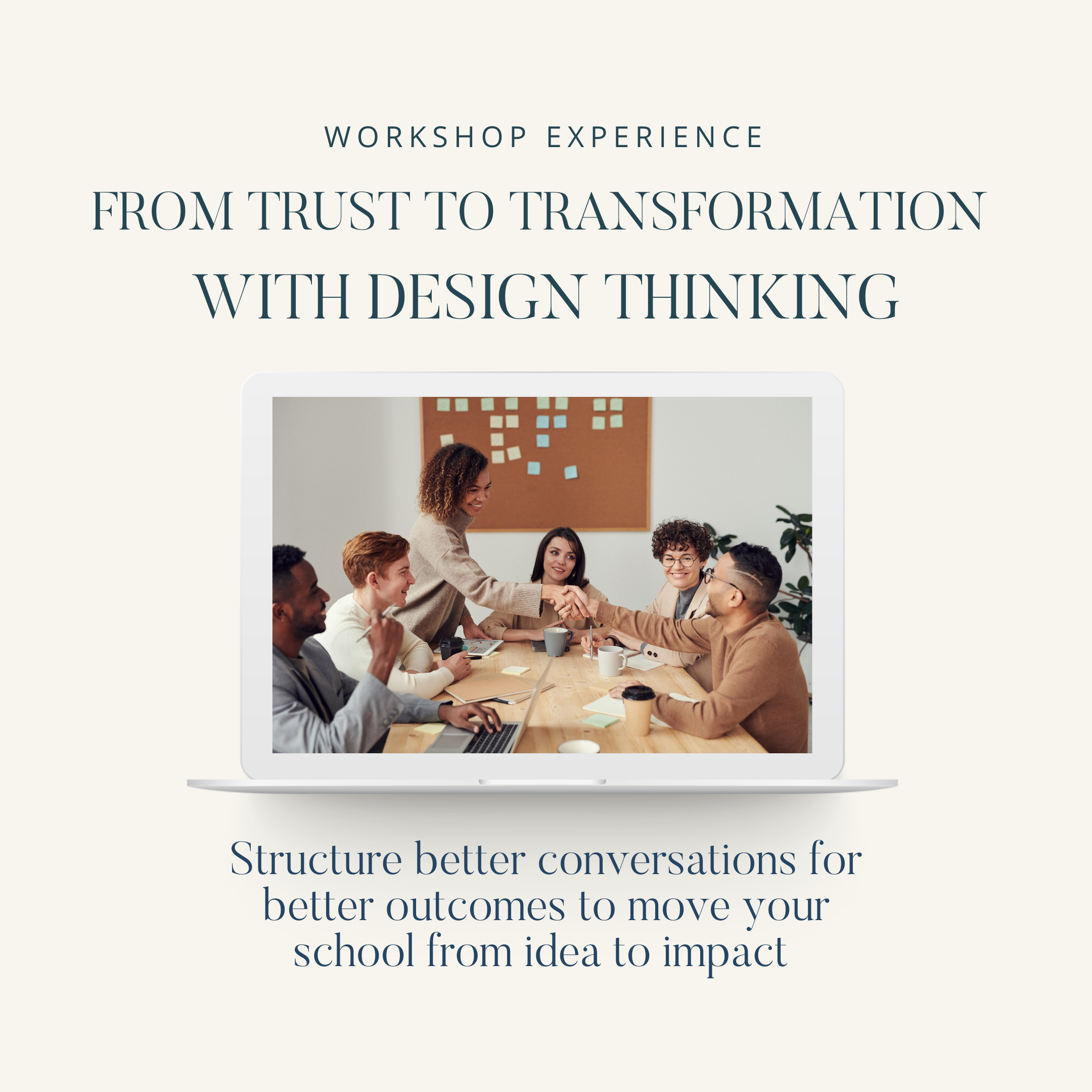
Attewell, P. (2001). The first and second digital divides. Sociology of Education, 74(3), 252-259
Borko, H., & Putnam, R.T. (1995). Expanding a teacher’s knowledge base: A cognitive psychological perspective on professional development. In T. Gusky & M. Huberman (Eds), Professional development in education: New paradigms and practices (pp.35-65). Teachers College Press.
Brown, T & Wyatt, J. (2010). Design thinking for social innovation. Stanford Social Science Review, 8 (1), 30-35.
Brynjolfsson, E. (2014). The second machine age: Work, progress, and prosperity in a time of brilliant technologies (1st t ed.). W. W. Norton & Company.
Carroll, M., Goldman, S., Britos, L., Koh, J., Royalty, A., & Hornstein, M. (2010). Destination, imagination and the fires within: Design thinking in a middle school classroom. International Journal of Art and Design Education, (29)1, 37-53.
Chin, D. B., Doris, Blair, K.P., Wolf, R., & Conlin, L., Cutumisu, M., Pfaffman, J., Schwartz, D.L. (2019). Educating and measuring choice: A test of the transfer of design thinking in problem solving and learning. Journal of the Learning Sciences. 1-44.
Levy, F., & Murnane, R. (2013). Dancing with Robots. NEXT Report.
McKinsey Global Institute (2017). Technology, Jobs and the Future of Work. McKinsey.
PwC (2017). Technology in U.S. Schools: Are we preparing our students for the jobs of tomorrow . Pricewater House Coopers. https://www.pwc.com/us/en/about-us/corporate-responsibility/library/preparing-students-for-technology-jobs.html .
Robinson, K., & Aronica, L. (2016). Creative schools: the grassroots revolution that’s transforming education. Penguin Books.
Shively, K., Stith, K.M., & Rubenstein, L.D. (2018). Measuring what matters: Assessing creativity, critical thinking, and the design process. Gifted Child Today, 41(3) 149-158.
World Economic Forum. (2018). The future of jobs: Employment, Skills and Workforce Strategy for the Fourth Industrial Revolution . World Economic Forum.
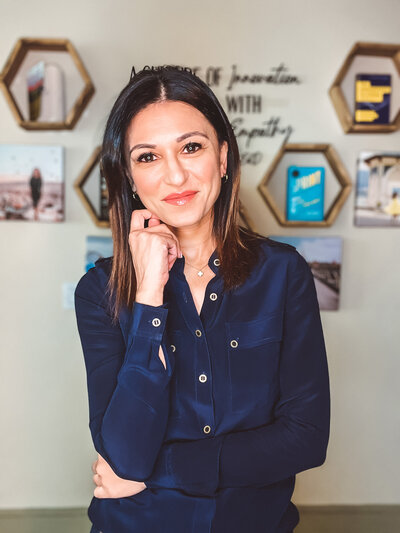
I believe that the future should be designed. Not left to chance. Over the past decade, using design thinking practices I've helped schools and businesses create a culture of innovation where everyone is empowered to move from idea to impact, to address complex challenges and discover opportunities.
stay connected
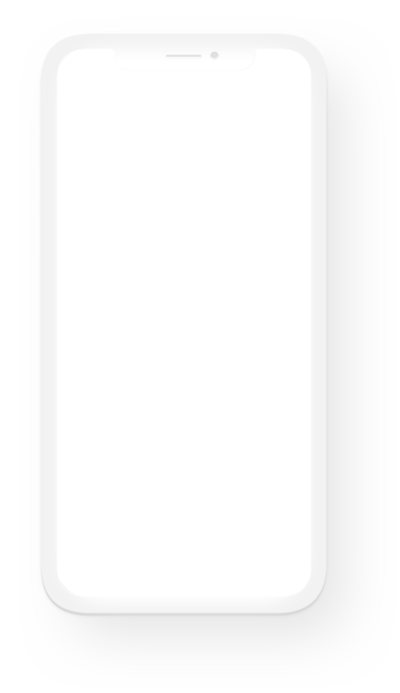
designing schools
[…] importance of learning experiences at the intersection of developing learning skills and mindsets. Design thinking is one approach that can help you master both. In my experience it’s rare that design thinking […]
Leave a Reply Cancel reply
Your email address will not be published. Required fields are marked *
Save my name, email, and website in this browser for the next time I comment.
This site uses Akismet to reduce spam. Learn how your comment data is processed .
« How Education Leaders Use Social Media to Build Trust, Encourage Creativity, and Inspire a Collective Vision
Creative Career Map: How Students Can Navigate the Future of Work with Design Thinking »
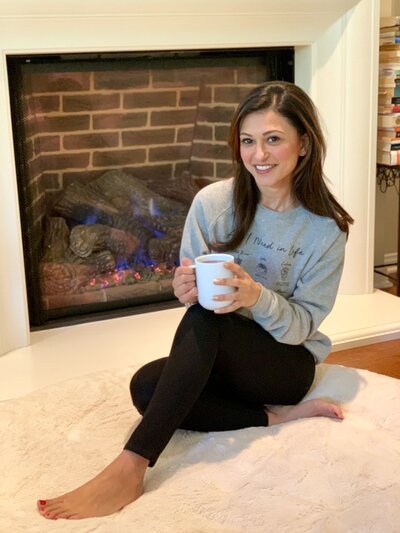
Browse By Category
Social Influence
Design Thinking
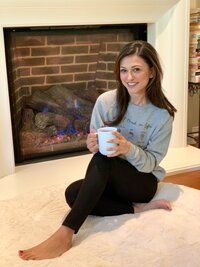
Join the Community
All of my best and life changing relationships began online. Whether it's a simple tweet, a DM or an email. It always begins and ends with the relationships we create. Each week I'll send the skills and strategies you need to build your human advantage in an AI world straight to your inbox.
As Simon Sinek Says: "Alone is hard. Together is better."
©DesigningSchools. All rights reserved. 2023
Instagram is my creative outlet. It's where you can see stories that take you behind the scenes and where I love having audio chats in my DM.
@designing_schools

Design Thinking has been widely recognized as an alternative way of thinking and methodology for problem solving in Education institutions from K-12 to Grad Schools in educational institutions such as Stanford, MIT, Darden School of Business at Virginia University, Carnegie Mellon and many more. Read about their courses, programs, research and other involvement with design thinking on this page.
See our listing of courses and programs offered by all Universities and other institutions on the Education page .
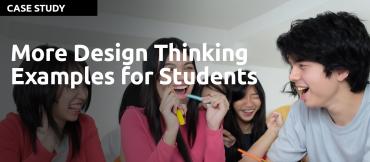
This is a follow on article to our original article "5 Design Thinking Examples for Students" .
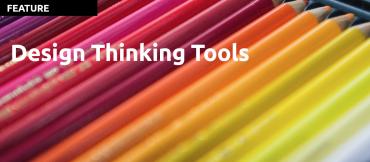
What are Design Thinking Tools? Design thinking is an approach to problem-solving that is centered around understanding the needs and experiences of end-users. It is a creative and innovative approach that is used by designers and organizations to develop effective solutions to complex problems. Design thinking tools are a set of methods and techniques that facilitate the design thinking process. In this article, we will discuss some of the most popular design thinking tools and their benefits.
1. Empathy Mapping
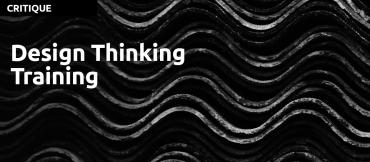
Design thinking training has become increasingly popular in recent years, with businesses and organizations around the world looking to develop their innovation and problem-solving capabilities. While design thinking can be a powerful tool for creativity and innovation, there are also some potential pitfalls and limitations to its use. In this article, we will critically examine design thinking training, looking at its strengths and weaknesses, and providing recommendations for how to make the most of this approach.
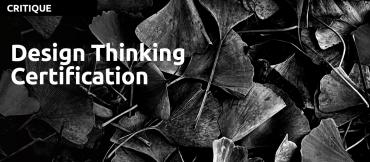
Design thinking has emerged as one of the most popular frameworks for creative problem-solving. It has been used by businesses, startups, and non-profits around the world to develop innovative solutions to complex challenges. As a result, the demand for design thinking certification has increased significantly. However, the question remains, is design thinking certification necessary, and does it truly add value to the process of design thinking?
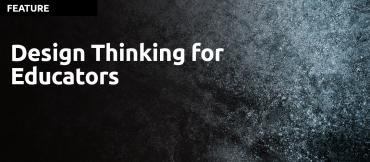
Welcome to our educators page. We hope that we can support you on your journey to discover and use Design Thinking to help the next generation to become more confident in using creativity to solve problems.
Why is Design Thinking important for students?
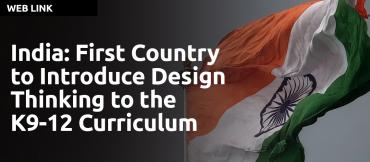
21st Century Learning and Innovation Skills are the skills that will be essential for students to possess to thrive in the increasingly complex life and work environments in the 21st century. These include:
• Creativity and Innovation • Critical Thinking and Problem Solving • Communication • Collaboration
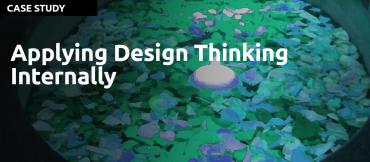
Applying Design Thinking internally, within a group, community or to ourselves. This is a new application of the Design Thinking Methodology.
An internal application in this sense can have two meanings. First, the internal application of design thinking tactics within a group, organization or community, and second, the internal application of design thinking to one’s own self and life.
Can Design Thinking help you solve your own problems?
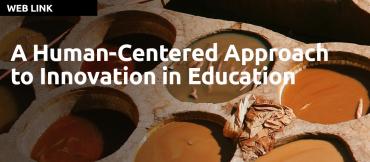
Talk to any educator, parent, or policy maker and you will inevitably hear about the many problems that exist in education. It’s not for lack of trying—millions of people are working across the country to find new solutions for our schools. And yet we’re struggling to find new answers that make a real difference.
Recently IDEO, the design and innovation firm where I work, collaborated with San Francisco Unified School District to develop a new vision for their food system.
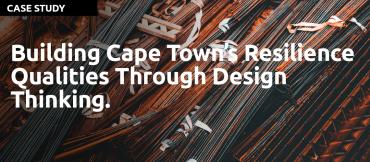
This case study focuses on a Design Thinking Workshop for primary school learners. The aim of the workshops was to provide learners with a new set of skills which they can employ when problem solving for real world challenges.
Building resilience is essential for cities that face increasing uncertainty and new challenges that threaten the well-being of its citizens. This is especially important when looking at the diversity and complexity of potential shocks and stresses.
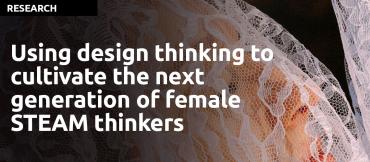
This study shows that a short, 3-day intervention can make a positive impact on young female youths’ perceptions of STEM, pro-social attitudes, creative confidence, and career pathways. It does this by creating a “hook” or stimulating interest among youths to have a more favorable opinion about working in STEM.
The study’s second finding is that persistent gender norms are hard to overturn and require additional interventions.
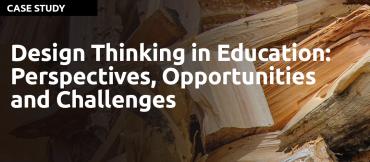
This very informative article discusses design thinking as a process and mindset for collaboratively finding solutions for wicked problems in a variety of educational settings. Through a systematic literature review the article organizes case studies, reports, theoretical reflections, and other scholarly work to enhance our understanding of the purposes, contexts, benefits, limitations, affordances, constraints, effects and outcomes of design thinking in education.
Specifically, the review pursues four questions:
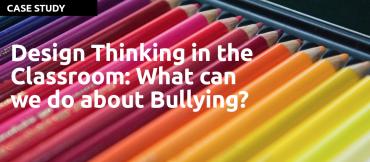
As children move from kindergarten, through middle school, and to high school, instruction shifts from stories to facts, from speculation to specifics, and imagination fades from focus. Design Thinking provides an alternative model to traditional ways of learning academic content by challenging students to find answers to complex, nuanced problems with multiple solutions and by fostering students’ ability to act as change agents.
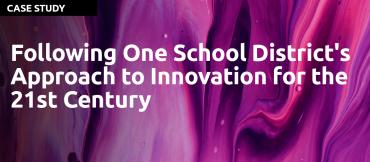
In her doctoral paper Loraine Rossi de Campos explores the use of Design Thinking in a school district for a 4-5 grade school.
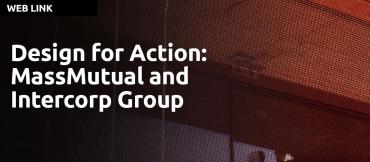
How to use design thinking to make great things actually happen by Tim Brown and Roger L. Martin. In this great HBR article, the authors look at design thinking in Finance with two case studies, one from MassMutual and the other from Intercorp. Group of Peru.
In this article highlighting the development of the acceptance of Design Thinking, they discuss how Design Thinking helps to create the artifact that creates the new solution as well as the intervention/s that brings the artifact to life.
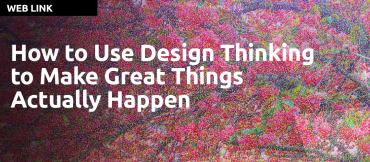
Ever since it became clear that smart design led to the success of many products, companies have been employing it in other areas, from customer experiences, to strategy, to business ecosystems. But as design is used in increasingly complex contexts, a new hurdle has emerged: gaining acceptance (for the new solutions).
Read more...
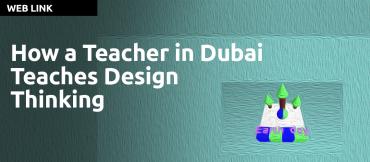
Reham has been working at Mohammed bin Rashid school, a public school in Dubai, for the last five years as a Design & Technology Teacher. She teaches grades 3 to grade 8 students. Prior to working as a teacher, Reham was an architect and worked at an engineering consultancy where her duties included designing buildings using Autocad and 3d Max software.
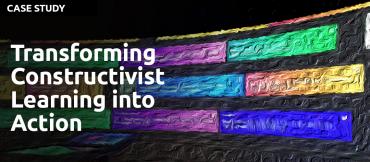
In an ever changing society of the 21st century, there is a demand to equip students with meta competences going beyond cognitive knowledge. Education, therefore, needs a transition from transferring knowledge to developing individual potentials with the help of constructivist learning. A Scheer, C Noweski, C Meinel , University of Potsdam, Germany.
Design Thinking is the most effective method of teaching constructivist learning.
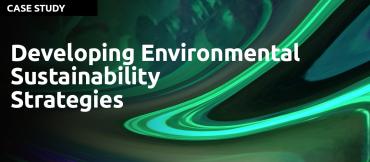
Developing environmental sustainability strategies, the Double Diamond method of LCA and design thinking: a case study from aged care. Journal of Cleaner Production, 85, 67-82. Stephen J. Clune*, Simon Lockrey.
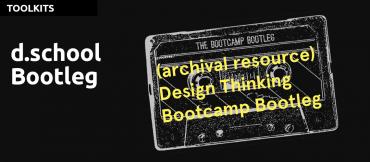
The Stanford University d.school - Design Thinking Bootcamp Bootleg , Design Thinking tools and the Resources page . have gone through a number of iterations to refine the exercises and tools. The Bootleg offers the latest version (as of May 2020) for people to download and use for free.
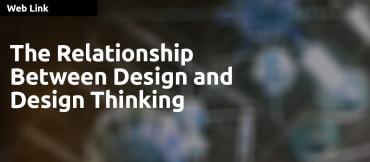
Watch this video from Nathan Shedroff on design strategy and the merging of business and design. In this video Nathan very clearly articulates the difference between design and design thinking and discusses how design needs to mesh with business and business with design. Well worth watching.
Watch Video...
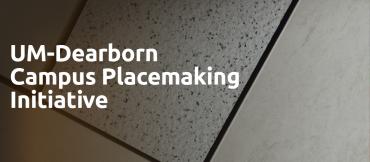
Admissions needs to communicate consistently at each touchpoint. Prospective students and families want to assess whether this educational experience is the right fit for them. Current students and alumni are asking for Maize and Blue landmarks to build the Michigan Tradition across campus.
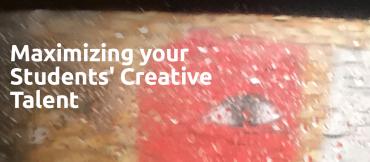
Design Thinking is a powerful approach to problem solving and supports schools in moving toward a more hands-and minds-on, challenge-based curriculum. We want kids to be engaged right? You won't believe how well Design Thinking engages kids.
Watch this video...
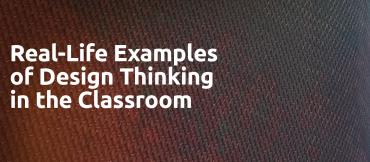
Design Thinking in daily life is about creating creative and collaborative workflows engineered to tackle big projects and prototyping to discover new solutions.
And although we have K-12 schools incorporating design thinking into their curriculum and instruction, as well as educators attending design thinking workshops at places like the d. School at Stanford, what does design thinking in daily life really look like in K-12 classrooms and schools?
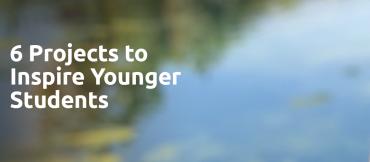
If we want our students to think critically, consider the needs of others and then develop solutions to solve real problems, design thinking project ideas offer a useful framework to make that happen. Are you looking to give it a try this year? Here are 6 design thinking project ideas that take students through the 5 stages – empathize, define, ideate, prototype and test – and get them digging deeper.
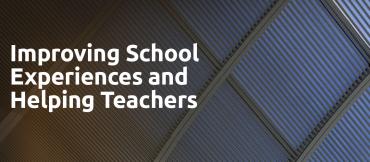
This video showcases three projects during this year’s Design Thinking Club. Each group of students used the design thinking method to help improve a school experience or help a teacher in need.
David Lee walks us through three Design Thinking projects. It's a great example of how the process works and the type of results that it produced.
Watch the kids go through the process and produce really great solutions. It is inspiring.
Watch here...
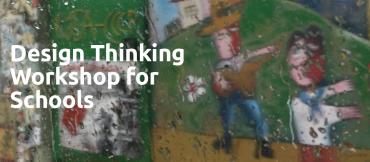
In this presentation from the Hathaway Brown School in Ohio, teachers will learn about the design thinking process, how it ties to the 21st century skills and how to conduct workshops for different grades. It provides a good overview for teachers who want to try Design Thinking in their classroom.

There are not that many Design Thinking project examples for students on the web. Most design thinking project examples are aimed at working professionals i.e. engineers, marketing, business people, and are not really great project examples for K-12 and college students to grasp how the process works. Nor is it easy to understand how they apply to your particular problem or passion.
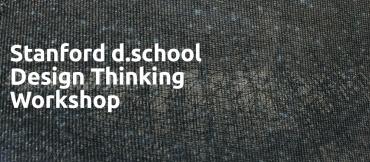
The Stanford d.school's Design Thinking Workshop is a powerful tool for developing innovative solutions to complex problems. The workshop combines empathy, creativity, and critical thinking to develop unique and effective solutions to a wide range of problems. This article will explore the how long the workshop should take, and some tips and tools to ensure a successful Design Thinking Workshop.
How Long Should the Workshop Take?
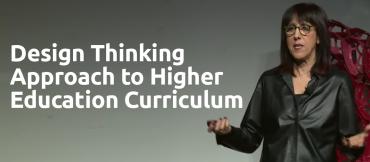
The way we are educating students is not the way that they will have to work to succeed in the world. When last did you see students working together in teams and collaborating on problem solving? Education is an individual pursuit in a world that needs team thinking and collaborative skills.
Listen to her TED Talk...
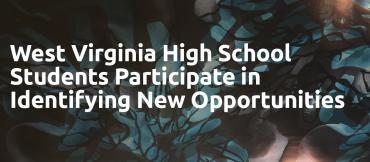
Too often, we hear students say they want to leave West Virginia because there are limited opportunities and few things to do. Simulated Workplace Entrepreneurship Education Pathway (SWEEP) turns that notion on its head and empowers students to generate and create those opportunities
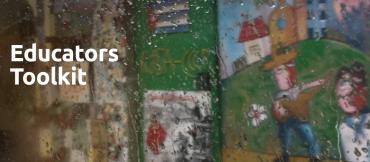
Design Thinking is a creative process that helps you design meaningful solutions to problems in the classroom, at your school, and in your community. This Design Thinking toolkit and Designer's Workbook provides you with instructions to explore Design Thinking with your students. Using the Design Thinking toolkit will help you to get your students engaged in the classroom in ways that are hard to achieve through other exercises.
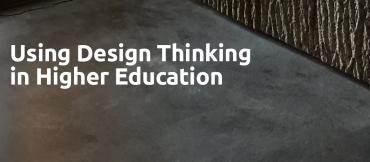
Design thinking offers a creative yet structured approach for addressing large-scale challenges.
We offer a summary of the webinar, Design Thinking: Education Edition , which discusses design thinking principles and process, describes real-world examples of design thinking in action, and offeres possibilities for how you might introduce the approach into your own organization.
- Open access
- Published: 05 September 2024
Exploring instructional design in K-12 STEM education: a systematic literature review
- Suarman Halawa 1 ,
- Tzu-Chiang Lin 2 , 3 &
- Ying-Shao Hsu ORCID: orcid.org/0000-0002-1635-8213 4
International Journal of STEM Education volume 11 , Article number: 43 ( 2024 ) Cite this article
1 Altmetric
Metrics details
This study aimed to analyze articles published in the Web of Science database from 2012 to 2021 to examine the educational goals and instructional designs for STEM education. We selected articles based on the following criteria: (a) empirical research; (b) incorporating instructional design and strategies into STEM teaching; (c) including intervention; (d) focusing on K-12 education and on assessment of learning outcomes; and (e) excluding higher education and STEAM education. Based on the criteria, 229 articles were selected for coding educational goals and instructional designs for STEM education. The aspects of STEM educational goals were coded including engagement and career choice, STEM literacy, and twenty-first century competencies. The categories of instructional designs for STEM education were examined including design-based learning, inquiry-based learning, project-based learning, and problem-based learning. The results showed that engagement and career choices and STEM literacy were mainly emphasized in STEM education. Design-based learning was adopted more than inquiry-based, project-based, or problem-based learning, and this instructional design was mainly used to achieve STEM literacy. It is suggested that studies on twenty-first century competencies may require more research efforts in future STEM education research.
Introduction
Emphasizing STEM (science, technology, engineering, and mathematics) has been the main focus of policy makers in many countries (English, 2016 ; National Academy of Engineering & National Research Council, 2014 ; National Research Council, 2012 , 2013 ) to meet economic challenges (Kelley & Knowles, 2016 ). Educational systems are accordingly prioritizing STEM to prepare students’ capability for the workplace to face the sophisticated technologies and competitive economy (Kayan-Fadlelmula et al., 2022 ). Hence, students are expected to be interested in STEM so that they will engage in and pursue careers in STEM-related fields (Lie et al., 2019 ; Struyf et al., 2019 ). Besides, we need a new generation that has the abilities to develop proficient knowledge, to apply such knowledge to solve problems, and to face existing and upcoming issues of the twenty-first century (Bybee, 2010 ).
Although STEM education has been proved to benefit students, there is a lack of understanding of instructional design for STEM education, despite the fact that such understanding is critical to research and to classroom practices. Limited understanding of relevant instructional design may lead to problems in implementing STEM education in the classroom. There is hence a need to examine educational goals, specific designs, and features of the instructional designs consistently and specifically documented in the STEM education literature. Therefore, this current study conducted systematic analysis of the literature to understand the educational goals and instructional designs for STEM education. Based on the analysis, we present a thorough picture of how researchers have developed instructional designs for STEM education.
Despite the fact that many researchers have promoted STEM education, the definition of STEM education has not reached a consensus in the literature, and there is a certain degree of disagreement in the scientific community. Lamb et al. ( 2015 ) defined STEM as a broad area encompassing many disciplines and epistemological practices. Other researchers, such as Breiner et al. ( 2012 ), defined STEM as applying transdisciplinary knowledge and skills in solving real-world problems. A similar definition established by Shaughnessy ( 2013 ) regarding STEM education is problem solving based on science and mathematics concepts that incorporate engineering strategies and technology. Another study defined STEM education as teaching approaches based on technology and engineering design that integrate the concepts and practices of science and mathematics (Sanders & Wells, 2006 ). In this study, we clarify STEM education as an approach that utilizes integrations of knowledge and skills from science, technology, engineering, and/or mathematics to solve real-world problems that help students to succeed in school learning, future careers, and/or society.
The definition of STEM as an integrated approach involving science, technology, engineering, and mathematics raises several pertinent questions about its composition and expectations. First, the requirement for all four disciplines to be present in order to qualify an educational program or project as “STEM” is debatable. Conceptually, integrating any two or more fields helps foster the interdisciplinary learning that is the hallmark of STEM education. This flexibility allows educators to tailor their programs to match the available resources and specific learning outcomes without necessarily incorporating all four disciplines in every instance. Regarding the classification of “science” within STEM, it is more a conglomerate of disciplines—such as biology, chemistry, physics, and earth sciences—than a single field. This diversity within science enriches STEM education, providing a broader knowledge base and problem-solving skills. Each scientific discipline brings a unique perspective and set of tools to the interdisciplinary mix, enhancing the complexity and richness of STEM learning experiences.
Furthermore, previous studies have identified several challenges to the implementation of STEM education in the classroom including poor motivation of students, weak connection with individual learners, little support from the school system, poor content without integration across disciplines, lack of quality assessments, poor facilities, and lack of hands-on experience (Ejiwale, 2013 ; Hsu & Fang, 2019 ; Margot & Kettler, 2019 ). To help teachers face challenges in the advancement of STEM education, Hsu and Fang ( 2019 ) proposed a 5-step STEM curriculum designs framework and provided examples of how to apply it to a lesson plan to help teachers design their instruction. This previous study also suggested that researchers conduct more investigations related to instructional design to enrich our understanding of various aspects of STEM education. Teachers of STEM require more opportunities to construct their perspective and a vision of STEM education as well as to conduct appropriate instructional designs. Moreover, from review articles published from 2000 to 2016, Margot and Kettler ( 2019 ) found that in multiple studies concerning similar challenges and supports, teachers believed that the availability of a quality curriculum would enhance the success of STEM education. Teachers need to provide and use an appropriate instructional design for STEM education and understand the educational goals. Therefore, we see the need to conduct research related to STEM education, especially exploring the instructional design because identifying and using a quality instructional design could increase the effectivess of STEM education.
According to the previous literature review, educational goals for instructional design were highlighted in STEM education. First, engagement and career choice need to be emphasized in STEM learning to improve students’ interest and self-efficacy (Vongkulluksn et al., 2018 ). Students need to engage in STEM education to raise their interest and engagement in STEM and to increase and develop a STEM-capable workforce (Honey et al., 2014 ; Hsu & Fang, 2019 ; Schütte & Köller, 2015 ). Engaging students in STEM education could improve their attitudes (Vossen et al., 2018 ) and their interest in STEM fields, and encourage them to pursue STEM careers (Means et al., 2017 ).
Second, STEM literacy needs to be promoted in K-12 schools (Falloon et al., 2020 ; Jackson et al., 2021 ) to develop students’ ability to encounter global challenges (Bybee, 2010 ). Students need to have the ability to apply concepts from science, technology, engineering, and mathematics, and skills to solve problems related to social, personal, and global issues in society (Bybee, 2010 ; Jackson et al., 2021 ). Besides, improving students’ STEM literacy is needed for their decision-making, participation in civic and cultural affairs, and economic productivity (National Academy of Engineering & National Research Council, 2014 ; National Research Council, 2011 ).
Last, regarding the twenty-first century competencies, students are anticipated to have abilities of creativity and innovation, problem solving, critical thinking, collaboration and communication (Boon, 2019 ) as citizens, workers, and leaders in the twenty-first century (Bryan et al., 2015 ; National Academy of Engineering & National Research Council, 2014 ; Stehle & Peters-Burton, 2019 ). These abilities are critical for students to adapt and thrive in a changing world (National Research Council, 2013 ). Also, students need to have the abilities to adapt to the twenty-first century in order to succeed in the new workforce (Bybee, 2013 ).
Considering the achievement of students’ engagement, motivation, STEM literacy, as well as twenty-first century competencies, many countries have significantly enlarged the funding for research and education relevant to STEM (Sanders, 2009 ). One of the strands of the existing research is to help teachers know how to implement STEM education in schools (Aranda, 2020 ; Barak & Assal, 2018 ; English, 2017 ). Researchers have proposed instructional designs for STEM education including design-based learning (Kelley & Knowles, 2016 ; Yata et al., 2020 ), inquiry-based learning (Bybee, 2010 ), project-based learning (Capraro et al., 2013 ), and problem-based learning (Carpraro & Slough, 2013 ).
Design-based learning focuses on technological and engineering design. This instructional design engages students in learning about engineering design practices (Fan et al., 2021 ; Guzey et al., 2016 ; Hernandez et al., 2014 ) through the steps of designing, building, and testing (Yata et al., 2020 ). Design-based learning promotes problem solving, design, building, testing, and communication skills (Johnson et al., 2015 ) and improves students’ interest in STEM activities (Vongkulluksn et al., 2018 ). Also, design-based learning improves students’ engineering abilities and twenty-first century competencies (Wu et al., 2019 ) and attitudes (Vossen et al., 2018 ), and engages them in understanding core disciplinary ideas (Guzey et al., 2016 ).
Inquiry-based learning focuses on engaging students in hands-on activities to investigate scientific phenomena (Lederman & Lederman, 2012 ) and to construct their new knowledge (Bybee, 2010 ; Halawa et al., 2020 ). Students are encouraged to plan and design their experiments, analyze and interpret data, argue, and communicate their findings (Halawa et al., 2023 ; National Research Council, 2012 , 2013 ). Inquiry-based learning is also deemed to improve students’ knowledge, interest, engagement (Sinatra et al., 2017 ) and creativity (Smyrnaiou et al., 2020 ). Besides, researchers have noticed the importance of inquiry-based learning for improving students’ attitudes toward science-related careers (Kim, 2016 ). Although inquiry-based learning mainly focuses on science education to engage students in authentic learning (Halawa et al., 2024 ), it has been known to share common goals and characteristics with mathematics, technology, and engineering (Grangeat et al., 2021 ; Lin et al., 2020 ). Common elements in STEM education are engaging students in asking questions and testing their ideas in a systematic and interactive way (Grangeat et al., 2021 ).
Project-based learning and problem-based learning, both instructional designs, engage students in experiential and authentic learning with open-ended and real-world problems (English, 2017 ). Yet, project-based learning tends to be of longer duration and occurs over an extended period of time (Wilson, 2021 ), while problem-based learning is usually embedded in multiple problems (Carpraro & Slough, 2013 ). STEM project-based learning focuses on engaging students in an ill-defined task within a well-defined outcome situated with a contextually rich task, requiring them to solve certain problems (Capraro et al., 2013 ). Project-based learning and problem-based learning are both used to develop students’ problem solving, creativity, collaboration skills (Barak & Assal, 2018 ), and attitude (Preininger, 2017 ).
According to previous studies, researchers have adopted STEM instructional designs to achieve certain educational goals. For instance, in the aspects of engagement and career choice, Sullivan and Bers ( 2019 ) used design-based learning to improve students’ interest in engineering and students’ performance in elementary school. Kang et al. ( 2021 ) adopted inquiry-based learning for secondary school by embedding careers education to foster the students’ interest in science. Vallera and Bodzin ( 2020 ) adopted project-based learning at primary school in the northeastern United States to improve students’ STEM literacy and attitude. Preininger ( 2017 ) used problem-based learning to influence students’ attitudes toward mathematics and careers involving mathematics. In the aspect of STEM literacy, King and English ( 2016 ) adopted design-based learning to enable students to apply STEM concepts to the model of the construction of an optical instrument. Han et al. ( 2015 ) adopted STEM project-based learning to improve the performance of low-performing students in mathematics. Lastly, regarding the twenty-first century competencies, English et al. ( 2017 ) adopted design-based learning to improve students’ capabilities of handling the complexity of the task (English et al., 2017 ).
In conclusion, studies have grown to explore educational goals related to instructional designs for STEM education. However, consistent and systematic reviews related to instructional designs in K-12 STEM education are comparatively scarce. Although there are some reviews of the STEM education literature (Andrews et al., 2022 ; Gladstone & Cimpian, 2021 ; Kaya-Fadlelmula et al., 2022 ; López et al., 2022 ; Margot & Kettler, 2019 ; Martín-Páez et al., 2019 ; Nguyen et al., 2021 ), it is noteworthy that previous studies only explored undergraduate instruction in STEM education (Andrews et al., 2022 ; Henderson et al., 2011 ; Nguyen et al., 2021 ). Therefore, to fill the research gap, this current study conducted a systematic analysis of literature to understand the educational goals and instructional designs for K-12 STEM education from articles published between 2012 and 2021. The research questions of this study were formulated as follows:
What STEM education goals were more focused on in the reviewed articles? What was the trend of educational goals in the reviewed articles?
What instructional designs were more focused on in the reviewed articles? What was the trend of the instructional design in the review articles?
What instructional designs were more focused on to achieve certain educational goals in the reviewed articles?
What features of instructional designs were more focused on in the reviewed articles?
Data collection
To identify the target literature for further analysis, this study conducted several rounds of searching the Web of Science (WOS) database for articles (Gough et al., 2012 ; Møller & Myles, 2016 ). A systematic literature review using the PRISMA guidelines was used for article selection (Møller & Myles, 2016 ). First, we searched for articles using the keyword “STEM Education” along with “learning”, “teaching”, “curriculum”, and “professional development”, to refine the search results. The search identified a total of 1,531 articles published in the Web of Science from 2012 to 2021 (Fig. 1 ). We initially excluded duplicated articles; the search retrieved a total of 1,513 articles. We then screened the titles, abstract, and keywords of the articles based on the following criteria: (a) empirical research; (b) incorporating instructional design and strategies into STEM teaching; (c) including intervention; (d) focusing on K-12 education and on assessment of learning outcomes; and (e) excluding higher education and STEAM education. During this screening, we discussed which articles met the criteria through round-table discussions, and determined the preliminary target candidates composed of 394 articles. A full-text examination was then conducted. In this round of examination, we removed the articles without clear information about the educational goals and instructional designs related to STEM education. Finally, a corpus of literature comprising 229 articles was formed for further analysis.
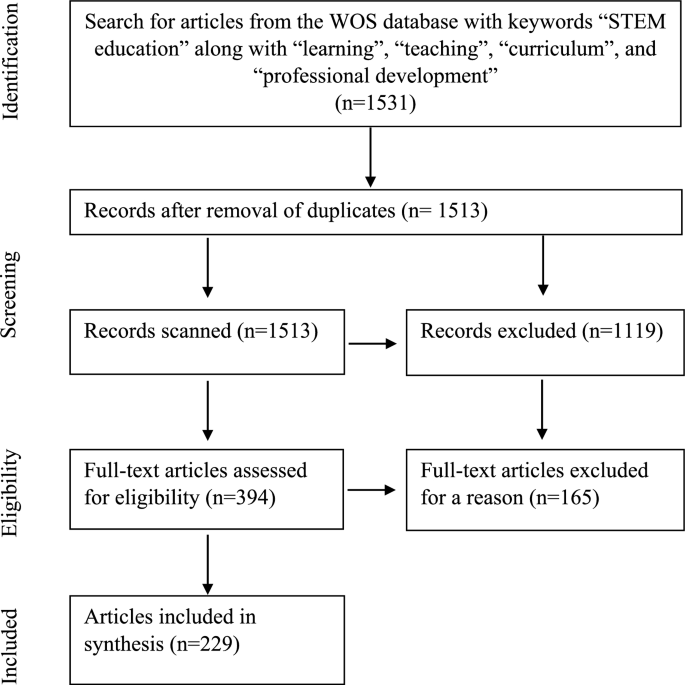
PRISMA flow diagram of articles selection
Data analysis
According to the research questions, for this study, we developed a coding framework to conduct content analysis and to categorize the target literature. We first selected paradigmatic references of STEM education and instructional design from high quality publications. These articles provided sets of core concepts and terms to shape the provisional coding categories. We then constantly reviewed the paradigmatic references and discussed them to improve the coding scheme. The final analytic framework with coding categories was developed as follows. The first category, STEM educational goals, includes engagement and career choice (Honey et al., 2014 ; Hsu & Fang, 2019 ), STEM literacy (Falloon et al., 2020 ; Jackson et al., 2021 ), and twenty-first century competencies (Boon, 2019 ) (see Appendix 1). The second category, instructional design, includes design-based learning (Yata et al., 2020 ), inquiry-based learning (Bybee, 2010 ; Halawa et al., 2020 ), project-based learning (Capraro & Slough, 2013 ), and problem-based learning (Priemer et al., 2020 ). From the review articles, we found that 6E - oriented STEM (engage, explore, explain, engineer, enrich, and evaluate) and game-based learning were used for STEM education. These two instructional designs were added to our coding scheme. Articles that did not specify the instructional design were coded as “others”. We then analyzed the outcomes to see whether instructional design successfully improved STEM educational goals. We analyzed design-based, inquiry-based, and project-based learning to achieve engagement and career choice, STEM literacy, and a combination of engagement and career choice and STEM literacy because the selected articles mainly concentrated on them. We categorized the outcomes as positively improved, partially improved, and none (Amador et al., 2021 ). Instructional design that successfully increased STEM educational goals was categorized as positively improved. Instructional design that only increased a part of STEM educational goals was categorized as partially improved. If the instructional design did not increase STEM educational goals, we categorized it as none.
We then extended our coding scheme to identify the features of design-based, inquiry-based, and project-based learning. We focused on these three instructional designs because the selected articles mainly adopted them. Yata et al. ( 2020 ) proposed designing, building, and testing as the features of design-based learning. Other features of instructional designs including questioning or identifying problems, experimenting, analyzing, explaining, collaborating, communicating, and reflecting were proposed as features of inquiry-based learning (Bybee, 2010 ; Halawa et al., 2020 ) and project-based learning (Capraro et al., 2013 ). From the review articles, we found that redesigning was one of the features of instructional design and so added it to the coding scheme. These features of instructional designs were adopted for our coding scheme including questioning or identifying problems, designing, building, testing, experimenting, analyzing, collaborating, reflecting, communicating, and redesigning (Appendix 2). We then calculated the number of articles that adopted these features of instructional designs. We further summarized the features of instructional designs that were frequently used in the selected articles.
In order to make sure the coding process was reliable, we conducted a trial coding by randomly selecting 40 articles and individually categorizing the articles into the aforementioned categories: (a) STEM education goal, and (b) instructional design. Interrater reliability was calculated using a percent agreement metric reaching an acceptable level of 0.85 (McHugh, 2012 ). The discrepancies between authors were negotiated and solved through discussions. The NVivo 11 software was utilized to complete coding works on the remaining articles. We then calculated and reported descriptive statistics of the coded data as the analytic results.
Engagement and career choice as the main focused STEM educational goals
Table 1 shows that more articles focused on engagement and career choice (64 articles) and STEM literacy (61 articles) than twenty-first century competencies (16 articles). The articles also mainly focused on a combination of engagement and career choice and STEM literacy (47 articles) and a combination of engagement and career choice and twenty-first century competencies (18 articles). Nine articles were found that focused on the three learning goals of engagement and career choice, STEM literacy, and twenty-first century competencies.
Table 1 shows the numbers of articles regarding educational goals for STEM education for each 2 years in the review papers. The number of articles per 2 years increased from 2012 to 2021. The trend analysis indicated that engagement and career choice and STEM literacy increased greatly from 2014 to 2021. The numbers of articles focused on the combination of two educational goals (STEM literacy and twenty-first competencies) and three learning goals (engagement and career choice, STEM literacy, and twenty-first competencies) from 2016 to 2021 are also presented.
Design-based and inquiry-based learning as the main instructional designs for STEM
Table 2 reveals the numbers of articles that used instructional design for STEM education. The instructional designs of design-based, inquiry-based, project-based, and problem-based learning were mainly used and continued to be used over the study period. The trend analysis indicated a big jump in design-based, inquiry-based, and project-based learning from 2018 to 2021.
Table 2 also shows the instructional designs and educational goals for STEM from review papers. Most articles adopted design-based (80 articles), inquiry-based (46 articles), project-based (42 articles), and problem-based (27 articles) learning.
Design-based learning mainly used to achieve STEM literacy
The findings shown in Table 3 identified that STEM instructional designs were used differently to achieve engagement and career choice, STEM literacy, and the combination of engagement and career choice and STEM literacy. We found that design-based learning was mainly adopted to achieve STEM literacy (28 articles), while inquiry-based learning was mainly used to achieve engagement and career choice (14 articles) and the combination of engagement and career choice and STEM literacy (14 articles). Also, more articles (15 articles) adopted project-based learning to achieve engagement and career choice. Furthermore, more design-based learning (7 articles) and problem-based learning (4 articles) than inquiry-based learning (2 articles) and project-based learning (1) articles were adopted to achieve twenty-first century competencies.
As we identified that a major portion of the articles adopted design-based learning, inquiry-based learning, and project-based learning focused on engagement and career choice, STEM literacy, and a combination of engagement and career choice and STEM literacy (see Table 3 ), we focused further analysis on the outcomes of STEM educational goals in the articles. The total number of selected articles was 124, of which 54 adopted design-based learning, 37 adopted inquiry-based learning, and 33 adopted project-based learning (Table 4 ).
We categorized the outcomes of STEM education goals into three categories (positively improved, partially improved, and none) (Amador et al., 2021 ). Table 4 shows that the majority of selected articles adopted design-based, inquiry-based, and project-based learning, improving STEM educational goals positively. Most selected articles found that design-based learning positively improved engagement and career choice (10 articles), STEM literacy (26 articles), and a combination of engagement and career choice and STEM literacy (15 articles). Also, most of the selected articles indicated that inquiry learning has a positive impact on engagement and career choice (14 articles), STEM literacy (7 articles), and a combination of engagement and career choice and STEM literacy (13 articles). Project-based learning has demonstrated a beneficial impact on various outcomes, as reported across the selected literature. Specifically, 12 articles documented the enhancement of engagement and career decisions, nine indicated the advancement of STEM literacy, and six discussed a combined effect on engagement, career choice, and STEM literacy.
Frequently used features of STEM instructional designs
To identify the frequently used features of STEM instructional design, we further explored the activities in the selected articles. As previous results show that the major part of articles adopted design-based learning, inquiry-based learning, and project-based learning, we further analyzed the frequently used features of these STEM instructional designs that focused on engagement and career choice, STEM literacy, and combination of engagement and career choice and STEM literacy (see Table 3 ). We selected 54 articles that adopted design-based learning, 37 adopted inquiry-based learning, and 33 adopted project-based learning (Table 5 ).
Frequently used features of design-based learning
Based on the findings, a large portion of the selected articles adopted design-based learning for STEM education (54 articles). Table 5 shows the features that were adopted to implement instructional design for design-based learning. More than half of the selected articles adopted designing, building, testing, collaborating, experimenting, and reflecting. Building (88.9%), designing (87.0%), and testing (70.4%) were used to engage students in engineering (Yata et al., 2020 ). Besides, engaging students in these activities required students to use their knowledge and skills (Kelley & Knowles, 2016 ). For example, Aranda et al. ( 2020 ) and Lie et al. ( 2019 ) implemented design-based learning by asking students to design a process to both prevent and test for cross-pollination of non-GMO from GMO fields. In these selected articles, the curriculums were focused on helping students with designing, building, and testing.
Collaborating, which engages students in working with their classmates in the process of design-based learning, was also mainly emphasized in the selected articles (64.8%). For instance, English and King ( 2019 ) asked students to work with their groups to discuss the possible design of the bridge. Researchers also emphasized experimenting (53.7%) to engage students in design-based learning. English ( 2019 ) engaged students in investigating their feet and shoes. Students collected, represented, analyzed data, and drew conclusions from their findings. Lie et al. ( 2019 ) helped students conduct an investigation to prevent cross-contamination of non-GMO from GMO corn fields. The last critical feature of design-based learning is reflecting (51.9%). In this activity, students engaged in assessing their solutions against a set of criteria and constraints, generating, and evaluating solutions (Cunningham et al., 2019 ). By engaging students in reflecting, students have an opportunity to improve their design and choose their best strategy (Aranda et al., 2020 ; Lie et al., 2019 ).
Frequently used features of inquiry-based learning
As shown in Table 5 , the inquiry-based learning approach was frequently adopted by researchers for STEM education. The features of this approach applied to achieve specific STEM education goals (e.g., engagement and career choice, and STEM literacy) included experimenting (91.9%), collaborating (83.8%), reflecting (62.2%), and communicating (51.4%) (see Table 5 ). This finding indicated that the top three frequently used features of inquiry-based learning in STEM were experimenting, collaborating, and reflecting, which play an essential role when learners try out their ideas about a real-world problem related to STEM. For example, a four-phase inquiry (clarifying the situation, hands-on experiments, representing, analyzing the produced data, and reporting/whole-class discussions) for authentic modeling tasks guided students to develop their credibility of the tasks and to acquire STEM knowledge (Carreira & Baioa, 2018 ).
Frequently used features of project-based learning
As previously mentioned, project-based learning is one of the major approaches to support instructional design in the reviewed STEM education studies. The results shown in Table 5 further indicate the features that researchers tended to integrate into instructional design for project-based learning. More than half (51.5%) of the selected articles reported “reflecting” as a pivotal part of teaching that triggered students’ project-based learning. Reflecting is deemed to depict learners’ active perceptions and deliberation of what they encounter and what they are doing. This may contribute to their competence to retrieve appropriate information, to provide feedback, and to revise the project underlying their learning. For example, in Dasgupta et al.’s ( 2019 ) study, a design journal was utilized to help students’ reflection on what they knew, what is necessary to know, as well as their learning outcomes. Vallera and Bodzin ( 2020 ) also addressed the critical design features of their curriculum to help students achieve information obtaining, evaluating, and communicating in the learning project based on real-world contexts.
Besides, researchers focused on project-based learning regarding STEM have a tendency to foster students’ learning via “identifying problems” (48.5%). These studies can be differentiated into two types based on whether the researchers provided a driving question for the learning project. In Vallera and Bodzin’s ( 2020 ) study, the instructional design arranged a clear-cut driving question to guide students’ thinking about helping farmers to prepare products for sale in a farmers’ market. This led students to extend their thinking and identify further problems while solving the driving question. As for Barak and Assal’s ( 2018 ) study, their instructional design provided open-ended tasks and ill-defined problems. Such arrangements were deemed to afford students’ learning through problem defining and learning objective setting.
It is also noteworthy to mention that the percentages of “experimenting” and “collaborating” in studies involved with project-based learning design were lower than those of studies with design-based learning or inquiry-based learning. However, researchers who were interested in STEM project-based learning would still to some extent agree with instructional design that may provide opportunities to students to access authentic scientific activities and social communications.
This study focused on analyzing the STEM educational goals and instructional designs adopted in the 2012–2021 articles. The findings of this study present knowledge and understanding of the educational goals that need to be considered in STEM education, and how these goals could be achieved by adopting various STEM instructional designs.
Educational goals for STEM education
The majority of reviewed articles adopted instructional designs to achieve the goals of engagement, career choice and STEM literacy. In contrast, few articles focused on twenty-first century competencies. It is not surprising because many recent studies in nature emphasized economic viewpoints and workplace-readiness outcomes in the STEM education field (Cheng et al., 2021 ; Kelley & Knowles, 2016 ). The aspects of engagement and career choice were frequently considered in many previous studies on STEM education (Struyf et al., 2019 ; Vongkulluksn et al., 2018 ; Vossen et al., 2018 ). It indicated that engagement and career choice are important goals for STEM education (Honey et al., 2014 ; Hsu & Fang, 2019 ; Kelley & Knowles, 2016 ). Engaging and motivating students in STEM education are necessary to enhance their understanding of their future careers (Fleer, 2021 ) and to cultivate them to continue STEM learning (Maltese et al., 2014 ). Students who were motivated and interested in STEM education would pursue STEM careers (Maltese & Tai, 2011 ). Furthermore, the aspects of STEM literacy are also addressed in the reviewed articles. The aspects of STEM literacy (e.g., knowledge and capabilities) are deemed important for students’ productive engagement with STEM studies, issues, and practices (Falloon et al., 2020 ). The focus of STEM literacy encourages students to apply their knowledge to life situations and solve problems (Bybee, 2010 ). The importance of STEM literacy has been highlighted in several national documents (e.g., Committee on STEM Education of the National Science & Technology Council, 2018 ; National Research Council, 2011 ; U.S. Department of Education, 2016 ). These findings provide insights into what teaching goals have been focused on in STEM education. For instance, engagement and career choice have been mainly focused on in STEM education because the STEM teaching was designed to connect to the students’ real-world experiences or future professional situations (Strobel et al., 2013 ). The authentic and meaningful experience could engage and motivate students in the activity, and later they should pursue their future careers related to what they have learned.
However, there are few selected articles focused on twenty-first century competencies, although many previous studies considered the twenty-first century competencies as important goals for students. Some studies have advocated that students should be engaged in interdisciplinary sets of complex problems and encourage them to use critical thinking and develop their creativity and innovation as well as collaboration (Finegold & Notabartolo, 2010 ; Jang, 2016 ). Engaging students in STEM education focused on twenty-first century competencies could prepare them for the workplace and help them become successful in STEM-related fields (Jang, 2016 ). Future researchers should consider integrating twenty-first century competencies into STEM education to complement the existing focus on engagement, career choice, and STEM literacy, preparing students for a broader range of skills necessary for the modern workforce.
Instructional design for STEM education
Although the reviewed articles adopted various instructional designs for STEM education, the articles mostly adopted design-based rather than inquiry-based, project-based, or problem-based learning. The findings are in accordance with the existing literature on STEM education. Notably, these results corroborate the conclusions drawn from a comprehensive systematic review conducted by Mclure et al. ( 2022 ). Design-based learning was adopted to achieve the goals of STEM literacy, engagement and career choice, and this instructional design tended to be used more often according to the trend analysis. This indicated that design-based learning was considered as a main instructional design for STEM education. This instructional design has become an essential approach to engaging K-12 students in STEM education (Bybee, 2013 ; National Academy of Engineering & National Research Council, 2014 ; National Research Council, 2013 ). Some researchers claimed that students who participate in design-based learning could make meaningful connections between knowledge and skills by solving problems (English & King, 2019 ; Kelley et al., 2010 ). Design-based learning engages students in authentic problems and challenges that increase their level of engagement (Sadler et al., 2000 ), help students learn fundamental scientific principles (Mehalik et al., 2008 ), and build students’ natural and intuitive experience (Fortus et al., 2004 ). In the process of design, students learn the concepts of science, technology, and mathematics in the process of designing, building, or testing products (Yata et al., 2020 ). For instance, students have to learn the concept of energy to design a house that produces more renewable energy than it consumes over a period of 1 year (Zheng et al., 2020 ). It was also found that the majority of selected articles which adopted design-based learning successfully improved learners’ engagement, career choice, and STEM literacy (Table 4 ). The results align with the findings of a previous meta-analysis focusing on STEM education at the middle school level (Thomas & Larwin, 2023 ). K-12 students’ STEM learning successfully improved because the selected articles reported studies conducting design-based learning in K-12 education. For example, Cunningham et al. ( 2019 ) successfully implemented design-based learning to improve elementary students’ learning outcomes, while Fan et al. ( 2018 ) found that design-based learning positively improved secondary students’ conceptual knowledge and attitude.
However, the selected articles have not equally used the features of design-based learning such as collaborating, reflecting, and redesigning. We identified that the selected articles mainly used designing, building, and testing to engage students in engineering activities. One of the explanations for this finding is that researchers may face challenges in implementing a full cycle of design-based learning because of the time limit of instruction, so they only focus on the process of designing, building, and testing. Collaborating, reflecting, and redesigning should be emphasized while adopting effective design-based learning because students could solve complex problems by collaborating with others. With collaboration, the students can learn/solve problems through discussion within the group. This activity allows students to share new ideas and debate with others to generate solutions. Reflecting on the data and experience allows students to make improvements to their model and leads them to redesign it to produce a better model. This process could also grow students’ science knowledge (Fortus et al., 2004 ). This finding hence suggests future studies, and educators emphasize more collaborating, reflecting, and redesigning for design-based learning for STEM instruction.
Moreover, inquiry-based learning, project-based learning, and problem-based learning were adopted in some selected articles. Inquiry-based learning was considered to enable and to promote connections within and across curriculum disciplines and improve students’ engagement in STEM education (Attard et al., 2021 ). Project-based and problem-based learning can be used to engage students in authentic problems (Blumenfeld et al., 1991 ) and to improve their engagement in STEM education (Beckett et al., 2016 ). Furthermore, we identified that inquiry-based learning mainly engages students in experimenting, collaborating, and reflecting (Kim, 2016 ), and project-based learning (Han et al., 2015 ) mainly engages students in identifying problems and reflecting. This finding reveals the frequently used features of inquiry-based learning and project-based learning. Teachers could use these components of instructional design for preparing their instruction for teaching STEM. Given these findings, it is advisable to explore the integration of inquiry-based, project-based, and problem-based learning alongside design-based learning in STEM education. Such an approach may enhance the effectiveness of STEM education by providing a more comprehensive strategy to improve STEM literacy, engagement, and career choice among K-12 students.
However, we identified that some essentials of these instructional designs have not been included in selected articles. For instance, studies adopting inquiry-based learning rarely asked students to propose their questions, although questioning is one of the frequently used features of inquiry (National Research Council, 2012 , 2013 ). One of the possible explanations for this finding is that students may have a lack of experience with inquiry learning and not know how to formulate meaningful questions, and they may tend to propose low-level factual questions related to their personal interests (Krajcik et al., 1998 ). Besides, STEM education requires students to engage in complex real-world problems, which requires sufficient ability to propose meaningful questions. Yet, we expect that future studies and teachers should encourage students to propose their own questions because questioning improves students’ creativity, critical thinking, and problem solving skills (Hofstein et al., 2005 ). Teachers could start asking students to propose their own questions once they have experience and ability to propose good questions. Krajcik et al. ( 1998 ) suggested providing situations in which students can receive informative and critical feedback from teachers, classmates, and others so as to propose their own significant questions.
Conclusions
From an instructional design perspective, this study provides crucial insights into practical STEM education approaches. The findings underscore the importance of aligning instructional designs with specific STEM educational goals. The trend analysis revealed a significant increase in focus on engagement, career choice, and STEM literacy from 2014 to 2021, with a particularly sharp rise observed between 2018 and 2021. Each instructional design approach demonstrated unique strengths: design-based learning fosters STEM literacy. In contrast, inquiry-based and project-based learning effectively enhanced engagement and career choice. The study delineates specific features of these instructional designs that contribute to their success, such as building and testing in design-based learning, experimenting and collaborating in inquiry-based learning, and reflecting and problem identification in project-based learning.
Furthermore, this study advocates for a deliberate and systematic application of inquiry-based and project-based learning alongside design-based learning. Such integration is likely to cultivate a more dynamic and interactive learning environment that encourages critical thinking, problem-solving, and collaborative skills among students. The integration of twenty-first century competencies in the instructional design of STEM, though less presented, suggests a potential research space for further exploration of STEM teaching. This study recommends an expanded focus on incorporating these competencies to ensure a holistic educational approach that addresses immediate educational goals and equips students with essential skills for future challenges.
Teachers’ limited understanding of STEM instructional design also presents a significant challenge, necessitating targeted professional development initiatives. Educators must comprehend and implement a comprehensive approach that aligns educational goals with appropriate instructional designs to optimize STEM learning outcomes. This approach involves clearly defining learning objectives, such as STEM literacy, selecting suitable instructional designs, and effectively guiding students through the chosen learning process.
The findings in this study furnish instructional designers and educators with a clear framework for developing targeted STEM curricula. The research accentuates the importance of aligning instructional design features with specific educational goals, suggesting that a nuanced, goal-oriented approach to STEM instruction can significantly enhance student outcomes in literacy, engagement, and career readiness. These insights offer a robust foundation for refining and optimizing instructional design strategies in STEM education.
Availability of data and materials
No applicable.
Amador, J. M., Bragelman, J., & Superfine, A. C. (2021). Prospective teachers’ noticing: A literature review of methodological approaches to support and analyze noticing. Teaching and Teacher Education . https://doi.org/10.1016/j.tate.2020.103256
Article Google Scholar
Andrews, T. C., Speer, N. M., & Shultz, G. V. (2022). Building bridges: A review and synthesis of research on teaching knowledge for undergraduate instruction in science, engineering, and mathematics. International Journal of STEM Education, 9 (1), 66. https://doi.org/10.1186/s40594-022-00380-w
Aranda, M. L., Guzey, S. S., & Moore, T. J. (2020). Multidisciplinary discourses in an engineering design-based science curricular unit. International Journal of Technology and Design Education, 30 (3), 507–529. https://doi.org/10.1007/s10798-019-09517-5
Attard, C., Berger, N., & Mackenzie, E. (2021). The positive influence of inquiry-based learning teacher professional learning and industry partnerships on student engagement with STEM. Frontiers in Education . https://doi.org/10.3389/feduc.2021.693221
Barak, M., & Assal, M. (2018). Robotics and STEM learning: Students’ achievements in assignments according to the P3 Task Taxonomy-practice, problem solving, and projects. International Journal of Technology and Design Education, 28 (1), 121–144. https://doi.org/10.1007/s10798-016-9385-9
Beckett, G. H., Hemmings, A., Maltbie, C., Wright, K., Sherman, M., & Sersion, B. (2016). Urban high school student engagement through cincySTEM iTEST projects. Journal of Science Education and Technology, 25 (6), 995–1007. https://doi.org/10.1007/s10956-016-9640-6
Berland, L., Steingut, R., & Ko, P. (2014). High school student perceptions of the utility of the engineering design process: Creating opportunities to engage in engineering practices and apply math and science content. Journal of Science Education and Technology, 23 (6), 705–720. https://doi.org/10.1007/s10956-014-9498-4
Blumenfeld, P. C., Soloway, E., Marx, R. W., Krajcik, J. S., Guzdial, M., & Palincsar, A. (1991). Motivating project-based learning: Sustaining the doing, supporting the learning. Educational Psychologist, 26 (3–4), 369–398. https://doi.org/10.1080/00461520.1991.9653139
Boon, N. S. (2019). Exploring STEM competences for the 21st century . Current and Critical Issues in Curriculum, Learning and Assessment 30. Geneva: IBEUNESCO. Retrieved June 19, 2022, from https://unesdoc.unesco.org/ark:/48223/pf0000368485.locale=en
Breiner, J. M., Harkness, S. S., Johnson, C. C., & Koehler, C. M. (2012). What is STEM? A discussion about conceptions of STEM in education and partnerships. School Science and Mathematics, 112 (1), 3–11. https://doi.org/10.1111/j.1949-8594.2011.00109.x
Bryan, L. A., Moore, T. J., Johnson, C. C., & Roehrig, G. H. (2015). Integrated STEM education. In C. C. Johnson, E. E. Peters-Burton, & T. J. Moore (Eds.), STEM roadmap: A framework for integration (pp. 23–37). Taylor & Francis.
Chapter Google Scholar
Bybee, R. W. (2010). Advancing STEM education: A 2020 vision. Technology and Engineering Teacher, 70 (1), 30–35.
Google Scholar
Bybee, R. W. (2013). The case for STEM education . NSTA press.
Capraro, R. M., Capraro, M. M., & Morgan, J. (Eds.). (2013). Project-based learning: An integrated science, technology, engineering, and mathematics (STEM) approach (2nd ed.). Sense.
Capraro, R. M., & Slough, S. W. (2013). Why PBL? Why STEM? Why now? an introduction to STEM project-based learning. In R. M. Capraro, M. M. Capraro, & J. R. Morgan (Eds.), STEM Project-based learning: An integrated Science, Technology, Engineering, and Mathematics (STEM) approach (pp. 1–5). Sense Publishers.
Carreira, S., & Baioa, A. M. (2018). Mathematical modelling with hands-on experimental tasks: On the student’s sense of credibility. ZDM-Mathematics Education, 50 (1–2), 201–215. https://doi.org/10.1007/s11858-017-0905-1
Chen, C. S., & Lin, J. W. (2019). A practical action research study of the impact of maker-centered STEM-PjBL on a rural middle school in Taiwan. International Journal of Science and Mathematics Education, 17 , S85–S108. https://doi.org/10.1007/s10763-019-09961-8
Cheng, L., Antonenko, P., Ritzhaupt, A. D., & MacFadden, B. (2021). Exploring the role of 3D printing and STEM integration levels in students’ STEM career interest. British Journal of Educational Technology, 52 (3), 1262–1278. https://doi.org/10.1111/bjet.13077
Committee on STEM Education of the National Science & Technology Council. (2018). Charting a course for success: America’s strategy for STEM education . Executive Office of the President National Science and Technology Council.
Cunningham, C. M., Lachapelle, C. P., Brennan, R. T., Kelly, G. J., Tunis, C. S. A., & Gentry, C. A. (2019). The impact of engineering curriculum design principles on elementary students’ engineering and science learning. Journal of Research in Science Teaching, 57 (3), 423–453. https://doi.org/10.1002/tea.21601
Dasgupta, C., Magana, A. J., & Vieira, C. (2019). Investigating the affordances of a CAD enabled learning environment for promoting integrated STEM learning. Computers & Education, 129 , 122–142. https://doi.org/10.1016/j.compedu.2018.10.014
Ejiwale, J. (2013). Barriers to successful implementation of STEM education. Journal of Education and Learning, 7 (2), 63–74.
English, L. D. (2016). STEM education K-12: Perspectives on integration. International Journal of STEM Education, 3 (1), 3. https://doi.org/10.1186/s40594-016-0036-1
English, L. D. (2017). Advancing elementary and middle school STEM education. International Journal of Science and Mathematics Education, 15 (1), 5–24. https://doi.org/10.1007/s10763-017-9802-x
English, L. D. (2019). Learning while designing in a fourth-grade integrated STEM problem. International Journal of Technology and Design Education, 29 (5), 1011–1032. https://doi.org/10.1007/s10798-018-9482-z
English, L. D., & King, D. (2019). STEM integration in sixth grade: Designing and constructing paper bridges. International Journal of Science and Mathematics Education, 17 (5), 863–884. https://doi.org/10.1007/s10763-018-9912-0
English, L. D., King, D., & Smeed, J. (2017). Advancing integrated STEM learning through engineering design: Sixth-grade students’ design and construction of earthquake resistant buildings. Journal of Educational Research, 110 (3), 255–271. https://doi.org/10.1080/00220671.2016.1264053
Falloon, G., Hatzigianni, M., Bower, M., Forbes, A., & Stevenson, M. (2020). Understanding K-12 STEM education: A framework for developing STEM literacy. Journal of Science Education and Technology, 29 (3), 369–385. https://doi.org/10.1007/s10956-020-09823-x
Fan, S.-C., Yu, K.-C., & Lin, K.-Y. (2021). A framework for implementing an engineering-focused stem curriculum. International Journal of Science and Mathematics Education, 19 (8), 1523–1541. https://doi.org/10.1007/s10763-020-10129-y
Fan, S.-C., Yu, K.-C., & Lou, S.-J. (2018). Why do students present different design objectives in engineering design projects? International Journal of Technology and Design Education . https://doi.org/10.1007/s10798-017-9420-5
Finegold, D., & Notabartolo, A. S. (2010). 21st century competencies and their impact: an interdisciplinary literature review. In D. Finegold, M. Gatta, H. Salzman, & S. J. Schurman (Eds.), Transforming the US workforce development system (pp. 19–56). Labor and Employment Relations Association.
Fleer, M. (2021). When preschool girls engineer: Future imaginings of being and becoming an engineer. Learning Culture and Social Interaction, 30 , 100372. https://doi.org/10.1016/j.lcsi.2019.100372
Fortus, D., Dershimer, R. C., Krajcik, J., Marx, R. W., & Mamlok-Naaman, R. (2004). Design-based science and student learning. Journal of Research in Science Teaching, 41 (10), 1081–1110. https://doi.org/10.1002/tea.2004
Gladstone, J. R., & Cimpian, A. (2021). Which role models are effective for which students? A systematic review and four recommendations for maximizing the effectiveness of role models in STEM. International Journal of STEM Education, 8 (1), 59. https://doi.org/10.1186/s40594-021-00315-x
Gough, D., Oliver, S., & Thomas, J. (2012). Introducing systematic reviews. In D. Gough, S. Oliver, & J. Thomas (Eds.), An introduction to systematic reviews (pp. 1–16). Sage.
Grangeat, M., Harrison, C., & Dolin, J. (2021). Exploring assessment in STEM inquiry learning classrooms. International Journal of Science Education, 43 (3), 345–361. https://doi.org/10.1080/09500693.2021.1903617
Guzey, S. S., Moore, T. J., Harwell, M., & Moreno, M. (2016). STEM integration in middle school life science: Student learning and attitudes. Journal of Science Education and Technology, 25 (4), 550–560. https://doi.org/10.1007/s10956-016-9612-x
Halawa, S., Hsu, Y.-S., & Zhang, W.-X. (2023). Analysis of physics textbooks through the lens of inquiry practices. The Asia-Pacific Education Researcher, 32 (4), 497–506. https://doi.org/10.1007/s40299-022-00671-4
Halawa, S., Hsu, Y.-S., & Zhang, W.-X. (2024). Inquiry activity design from Singaporean and indonesian physics textbooks. Science & Education, 33 (3), 581–607. https://doi.org/10.1007/s11191-022-00396-2
Halawa, S., Hsu, Y.-S., Zhang, W.-X., Kuo, Y.-R., & Wu, J.-Y. (2020). Features and trends of teaching strategies for scientific practices from a review of 2008–2017 articles. International Journal of Science Education, 42 (7), 1183–1206. https://doi.org/10.1080/09500693.2020.1752415
Han, S., Capraro, R., & Capraro, M. M. (2015). How science, technology, engineering, and mathematics (stem) project-based learning (pbl) affects high, middle, and low achievers differently: The impact of student factors on achievement. International Journal of Science and Mathematics Education, 13 (5), 1089–1113. https://doi.org/10.1007/s10763-014-9526-0
Henderson, C., Beach, A., & Finkelstein, N. (2011). Facilitating change in undergraduate STEM instructional practices: An analytic review of the literature. Journal of Research in Science Teaching, 48 (8), 952–984. https://doi.org/10.1002/tea.20439
Hernandez, P. R., Bodin, R., Elliott, J. W., Ibrahim, B., Rambo-Hernandez, K. E., Chen, T. W., & de Miranda, M. A. (2014). Connecting the STEM dots: Measuring the effect of an integrated engineering design intervention. International Journal of Technology and Design Education, 24 (1), 107–120. https://doi.org/10.1007/s10798-013-9241-0
Hofstein, A., Navon, O., Kipnis, M., & Mamlok-Naaman, R. (2005). Developing students’ ability to ask more and better questions resulting from inquiry-type chemistry laboratories. Journal of Research in Science Teaching, 42 (7), 791–806. https://doi.org/10.1002/tea.20072
Honey, M., Pearson, G., & Schweingruber, H. (Eds.). (2014). STEM integration in K-12 education: Status, prospects and an agenda for research . National Academies Press.
Hsu, Y.-S., & Fang, S.-C. (2019). Opportunities and challenges of STEM education. In Y.-S. Hsu & Y.-F. Yeh (Eds.), Asia-Pacific STEM teaching practices: From theoretical frameworks to practices (pp. 1–16). Springer Singapore.
Jackson, C., Mohr-Schroeder, M. J., Bush, S. B., Maiorca, C., Roberts, T., Yost, C., & Fowler, A. (2021). Equity-oriented conceptual framework for k-12 STEM literacy. International Journal of STEM Education, 8 (1), 38. https://doi.org/10.1186/s40594-021-00294-z
Jang, H. (2016). Identifying 21st century STEM competencies using workplace data. Journal of Science Education and Technology, 25 (2), 284–301. https://doi.org/10.1007/s10956-015-9593-1
Johnson, C. C., Peters-Burton, E. E., & Moore, T. J. (2015). STEM road map: A framework for integrated STEM Education . Routledge.
Book Google Scholar
Kang, J., Salonen, A., Tolppanen, S., Scheersoi, A., Hense, J., Rannikmae, M., & Keinonen, T. (2021). Effect of embedded careers education in science lessons on students’ interest, awareness, and aspirations. International Journal of Science and Mathematics Education . https://doi.org/10.1007/s10763-021-10238-2
Kayan-Fadlelmula, F., Sellami, A., Abdelkader, N., & Umer, S. (2022). A systematic review of STEM education research in the GCC countries: Trends, gaps and barriers. International Journal of STEM Education, 9 (1), 2. https://doi.org/10.1186/s40594-021-00319-7
Kelley, T. R., Brenner, D. C., & Pieper, J. T. (2010). Two approaches to engineering design: Observations in STEM education. Journal of STEM Teacher Education, 47 (2), 5–40.
Kelley, T. R., & Knowles, J. G. (2016). A conceptual framework for integrated STEM education. International Journal of STEM Education, 3 (1), 11. https://doi.org/10.1186/s40594-016-0046-z
Kim, H. (2016). Inquiry-based science and technology enrichment program for middle school-aged female students. Journal of Science Education and Technology, 25 (2), 174–186. https://doi.org/10.1007/s10956-015-9584-2
Krajcik, J., Blumenfeld, P. C., Marx, R. W., Bass, K. M., Fredricks, J., & Soloway, E. (1998). Inquiry in project-based science classrooms: Initial attempts by middle school students. Journal of the Learning Sciences, 7 (3–4), 313–350. https://doi.org/10.1080/10508406.1998.9672057
Lamb, R., Akmal, T., & Petrie, K. (2015). Development of a cognition-priming model describing learning in a STEM classroom. Journal of Research in Science Teaching, 52 (3), 410–437. https://doi.org/10.1002/tea.21200
Lederman, N. G., & Lederman, J. S., et al. (2012). Nature of scientific knowledge and scientific inquiry: Building instructional capacity through professional development. In B. J. Fraser (Ed.), Second international handbook of science education (pp. 335–359). Springer.
Lie, R., Guzey, S. S., & Moore, T. J. (2019). Implementing engineering in diverse upper elementary and middle school science classrooms: Student learning and attitudes. Journal of Science Education and Technology, 28 (2), 104–117. https://doi.org/10.1007/s10956-018-9751-3
Lin, K.-Y., Hsiao, H.-S., Williams, P. J., & Chen, Y.-H. (2020). Effects of 6E-oriented STEM practical activities in cultivating middle school students’ attitudes toward technology and technological inquiry ability. Research in Science & Technological Education, 38 (1), 1–18. https://doi.org/10.1080/02635143.2018.1561432
López, N., Morgan, D. L., Hutchings, Q. R., & Davis, K. (2022). Revisiting critical STEM interventions: A literature review of STEM organizational learning. International Journal of STEM Education, 9 (1), 39. https://doi.org/10.1186/s40594-022-00357-9
Maltese, A. V., Melki, C. S., & Wiebke, H. L. (2014). The nature of experiences responsible for the generation and maintenance of interest in STEM. Science Education, 98 (6), 937–962. https://doi.org/10.1002/sce.21132
Maltese, A. V., & Tai, R. H. (2011). Pipeline persistence: Examining the association of educational experiences with earned degrees in STEM among US students. Science Education, 95 (5), 877–907. https://doi.org/10.1002/sce.20441
Margot, K. C., & Kettler, T. (2019). Teachers’ perception of STEM integration and education: A systematic literature review. International Journal of STEM Education, 6 (1), 2. https://doi.org/10.1186/s40594-018-0151-2
Martín-Páez, T., Aguilera, D., Perales-Palacios, F. J., & Vílchez-González, J. M. (2019). What are we talking about when we talk about STEM education? A Review of Literature. Science Education, 103 (4), 799–822. https://doi.org/10.1002/sce.21522
McHugh, M. L. (2012). Interrater reliability: The kappa statistic. Biochemia Medica, 22 , 276–282.
McLure, F. I., Tang, K.-S., & Williams, P. J. (2022). What do integrated STEM projects look like in middle school and high school classrooms? A systematic literature review of empirical studies of iSTEM projects. International Journal of STEM Education, 9 (1), 73. https://doi.org/10.1186/s40594-022-00390-8
Means, B., Wang, H., Wei, X., Lynch, S., Peters, V., Young, V., & Allen, C. (2017). Expanding STEM opportunities through inclusive STEM-focused high schools. Science Education, 101 (5), 681–715. https://doi.org/10.1002/sce.21281
Mehalik, M. M., Doppelt, Y., & Schuun, C. D. (2008). Middle-school science through design-based learning versus scripted inquiry: Better overall science concept learning and equity gap reduction. Journal of Engineering Education, 97 (1), 71–85. https://doi.org/10.1002/j.2168-9830.2008.tb00955.x
Møller, A. M., & Myles, P. S. (2016). What makes a good systematic review and meta-analysis? BJA British Journal of Anaesthesia, 117 (4), 428–430. https://doi.org/10.1093/bja/aew264
National Academy of Engineering and National Research Council. (2014). STEM integration in K-12 education: Status, prospects, and an agenda for research . National Academies Press.
National Research Council. (2011). Successful K-12 STEM education: Identifying effective approaches in science, technology, engineering, and mathematics . National Academies Press.
National Research Council. (2012). A framework for K-12 science education: Practices, crosscutting concepts, and core ideas . The National Academies Press.
National Research Council. (2013). Monitoring progress toward successful K-12 STEM education: A nation advancing? National Academies Press. https://doi.org/10.17226/13509
Nguyen, K. A., Borrego, M., Finelli, C. J., DeMonbrun, M., Crockett, C., Tharayil, S., Shekhar, P., Waters, C., & Rosenberg, R. (2021). Instructor strategies to aid implementation of active learning: A systematic literature review. International Journal of STEM Education, 8 (1), 9. https://doi.org/10.1186/s40594-021-00270-7
Preininger, A. M. (2017). Embedded mathematics in chemistry: A case study of students’ attitudes and mastery. Journal of Science Education and Technology, 26 (1), 58–69. https://doi.org/10.1007/s10956-016-9651-3
Priemer, B., Eilerts, K., Filler, A., Pinkwart, N., Rösken-Winter, B., Tiemann, R., & Zu Belzen, A. U. (2020). A framework to foster problem-solving in STEM and computing education. Research in Science & Technological Education, 38 (1), 105–130. https://doi.org/10.1080/02635143.2019.1600490
Sadler, P. M., Coyle, H. P., & Schwartz, M. (2000). Engineering competitions in the middle school classroom: Key elements in developing effective design challenges. Journal of the Learning Sciences, 9 (3), 299–327. https://doi.org/10.1207/S15327809JLS0903_3
Sanders, M. (2009). STEM, STEM education, STEMmania. The Technology Teacher, 68 (4), 20–26.
Sanders, M. E., & Wells, J. (2006). Integrative STEM education course syllabi & instructional materials: STEM education foundations . In STEM Education Trends & Issues, STEM Education Seminar.
Schütte, K., & Köller, O. (2015). ‘Discover, understand, implement, and transfer’: Effectiveness of an intervention programme to motivate students for science. International Journal of Science Education, 37 (14), 2306–2325. https://doi.org/10.1080/09500693.2015.1077537
Shaughnessy, J. M. (2013). Mathematics in a STEM context. Mathematics Teaching in the Middle School, 18 (6), 324. https://doi.org/10.5951/mathteacmiddscho.18.6.0324
Sinatra, G. M., Mukhopadhyay, A., Allbright, T. N., Marsh, J. A., & Polikoff, M. S. (2017). Speedometry: A vehicle for promoting interest and engagement through integrated STEM instruction. Journal of Educational Research, 110 (3), 308–316. https://doi.org/10.1080/00220671.2016.1273178
Smyrnaiou, Z., Georgakopoulou, E., & Sotiriou, S. (2020). Promoting a mixed-design model of scientific creativity through digital storytelling—the CCQ model for creativity. International Journal of STEM Education, 7 (1), 25. https://doi.org/10.1186/s40594-020-00223-6
Stehle, S. M., & Peters-Burton, E. E. (2019). Developing student 21st Century skills in selected exemplary inclusive STEM high schools. International Journal of STEM Education, 6 (1), 39. https://doi.org/10.1186/s40594-019-0192-1
Strobel, J., Wang, J., Weber, N. R., & Dyehouse, M. (2013). The role of authenticity in design-based learning environments: The case of engineering education. Computers & Education, 64 , 143–152. https://doi.org/10.1016/j.compedu.2012.11.026
Struyf, A., De Loof, H., Boeve-de Pauw, J., & Van Petegem, P. (2019). Students’ engagement in different STEM learning environments: Integrated STEM education as promising practice? International Journal of Science Education, 41 (10), 1387–1407. https://doi.org/10.1080/09500693.2019.1607983
Sullivan, A., & Bers, M. U. (2019). Investigating the use of robotics to increase girls’ interest in engineering during early elementary school. International Journal of Technology and Design Education, 29 (5), 1033–1051. https://doi.org/10.1007/s10798-018-9483-y
Thomas, D. R., & Larwin, K. H. (2023). A meta-analytic investigation of the impact of middle school STEM education: Where are all the students of color? International Journal of STEM Education, 10 (1), 43. https://doi.org/10.1186/s40594-023-00425-8
U.S. Department of Education. (2016). STEM 2026: A vision for innovation in STEM education . U.S. Department of Education.
Vallera, F. L., & Bodzin, A. M. (2020). Integrating STEM with AgLIT (Agricultural literacy through innovative technology): The efficacy of a project-based curriculum for upper-primary students. International Journal of Science and Mathematics Education, 18 (3), 419–439. https://doi.org/10.1007/s10763-019-09979-y
Vongkulluksn, V. W., Matewos, A. M., Sinatra, G. M., & Marsh, J. A. (2018). Motivational factors in makerspaces: A mixed methods study of elementary school students’ situational interest, self-efficacy, and achievement emotions. International Journal of STEM Education, 5 (1), 43. https://doi.org/10.1186/s40594-018-0129-0
Vossen, T. E., Henze, I., Rippe, R. C. A., Van Driel, J. H., & De Vries, M. J. (2018). Attitudes of secondary school students towards doing research and design activities. International Journal of Science Education, 40 (13), 1629–1652. https://doi.org/10.1080/09500693.2018.1494395
Wilson, K. (2021). Exploring the challenges and enablers of implementing a STEM project-based learning programme in a diverse junior secondary context. International Journal of Science and Mathematics Education, 19 (5), 881–897. https://doi.org/10.1007/s10763-020-10103-8
Wu, Y., Guo, S., & Zhu, L. (2019). Design and implementation of data collection mechanism for 3D design course based on xAPI standard. Interactive Learning Environments, 28 (5), 602–619. https://doi.org/10.1080/10494820.2019.1696842
Yata, C., Ohtani, T., & Isobe, M. (2020). Conceptual framework of STEM based on Japanese subject principles. International Journal of STEM Education, 7 (1), 12. https://doi.org/10.1186/s40594-020-00205-8
Zheng, J., Xing, W., Zhu, G., Chen, G., Zhao, H., & Xie, C. (2020). Profiling self-regulation behaviors in STEM learning of engineering design. Computers & Education . https://doi.org/10.1016/j.compedu.2019.103669
Download references
Acknowledgements
The authors express their sincere gratitude to the editors and reviewers for their invaluable inputs and suggestions, which have significantly enhanced the quality of this work.
This work was financially supported by the Institute for Research Excellence in Learning Sciences of National Taiwan Normal University (NTNU) from The Featured Areas Research Center Program within the framework of the Higher Education Sprout Project by the Ministry of Education (MOE) in Taiwan.
Author information
Authors and affiliations.
Research Center for Education, National Research and Innovation Agency, Jakarta, Indonesia
Suarman Halawa
Center for Teacher Education, National Kaohsiung University of Science and Technology, Kaohsiung, Taiwan
Tzu-Chiang Lin
Center for the Liberal Arts, National Kaohsiung University of Science and Technology, Kaohsiung, Taiwan
Graduate Institute of Science Education, National Taiwan Normal University, Taipei, Taiwan
Ying-Shao Hsu
You can also search for this author in PubMed Google Scholar
Contributions
SH contributed to the conception of the study, research question, methods, analysis, and interpretation of the data. TC contributed to the data collection, analysis and interpretation of data, and editing of the manuscript. YS contributed to the conception of the study, data analysis and interpretation, and editing of the manuscript. All authors equally contributed to writing, reading, and approving the manuscript.
Corresponding author
Correspondence to Ying-Shao Hsu .
Ethics declarations
Ethics approval and consent to participate.
Not applicable.
Consent for publication
Competing interests.
The author declare that they have no competing interests.
Additional information
Publisher's note.
Springer Nature remains neutral with regard to jurisdictional claims in published maps and institutional affiliations.
Description of STEM education goals
STEM education goals | Brief description | Representational articles |
---|---|---|
Engagement and career choice | The goals of instruction focus on students’ emotional responses to learning STEM subjects and pursuing a professional degree in one of the STEM fields | Fan et al. ( ) |
STEM literacy | The goals of instruction focus on students’ ability to apply concepts from science, technology, engineering, and mathematics to solve problems that cannot be solved with a single subject | Vallera and Bodzin ( ) |
21st-century competencies | The goals of instruction focus on students’ abilities of critical thinking, creativity, innovation, leadership, and adaptability which can be used to adapt in the twenty-first century | Chen and Lin ( ) |
Description of the elements of instructional design for STEM education
Features | Brief description | Representational articles |
---|---|---|
Questioning or identifying problems | Students propose questions or identify problems in the STEM activity | Vallera and Bodzin ( ) |
Designing | Students design their model | Aranda et al. ( ) |
Building | Students build a prototype based on their model | English ( ) |
Testing | Students test their design and prototype | Zheng et al., |
Redesigning | Students redesign their model after they test it | Lie et al. ( ) |
Experimenting | Students engage in hands-on activities in the STEM education | Kim, |
Analyzing | Students use mathematics to analyze the data from the STEM activity | Berland et al. ( ) |
Collaborating | Students interact or collaborate with other students to solve problems in the STEM activity | English and King ( ) |
Reflecting | Students evaluate/assess their experience in the STEM activity | Dasgupta et al. ( ) |
Communicating | Students present/share their work to/with the whole class | Chen and Lin ( ) |
Rights and permissions
Open Access This article is licensed under a Creative Commons Attribution 4.0 International License, which permits use, sharing, adaptation, distribution and reproduction in any medium or format, as long as you give appropriate credit to the original author(s) and the source, provide a link to the Creative Commons licence, and indicate if changes were made. The images or other third party material in this article are included in the article's Creative Commons licence, unless indicated otherwise in a credit line to the material. If material is not included in the article's Creative Commons licence and your intended use is not permitted by statutory regulation or exceeds the permitted use, you will need to obtain permission directly from the copyright holder. To view a copy of this licence, visit http://creativecommons.org/licenses/by/4.0/ .
Reprints and permissions
About this article
Cite this article.
Halawa, S., Lin, TC. & Hsu, YS. Exploring instructional design in K-12 STEM education: a systematic literature review. IJ STEM Ed 11 , 43 (2024). https://doi.org/10.1186/s40594-024-00503-5
Download citation
Received : 15 April 2024
Accepted : 24 August 2024
Published : 05 September 2024
DOI : https://doi.org/10.1186/s40594-024-00503-5
Share this article
Anyone you share the following link with will be able to read this content:
Sorry, a shareable link is not currently available for this article.
Provided by the Springer Nature SharedIt content-sharing initiative
- STEM education
- Engagement and career choice
- STEM literacy
Introduction: Design Thinking in the Field of Education
- First Online: 13 April 2022
Cite this chapter
- Timm Krohn 3 &
- Christoph Meinel 4
1617 Accesses
1 Citations
Design Thinking has become an established approach in science and the commercial sector so that companies and institutions worldwide are benefiting from this new problem-solving and innovation mindset. At HPI, we have learned over the past 15+ years that design thinkers, whether students or professionals, develop a more thorough awareness of problems, take a user-focused approach to thinking and solving as a team, and achieve amazing results when working in groups. The expertise of individuals is bundled to form a group’s overall knowledge. If the process is moderated and focused, the team’s problem-solving power exceeds the sum of the available specialized knowledge. Thus, Design Thinking turns students into creative problem solvers and socially competent team workers. These skills are in demand and are increasingly required in managerial positions in the networked economy. HPI and its Design Thinking facilities continue to research this approach while educating and teaching students. In this book, researchers and practitioners from the HPI cosmos present their research approaches and results. We would like to share our experience of about 15 years of working in Design Thinking education.
This is a preview of subscription content, log in via an institution to check access.
Access this chapter
Subscribe and save.
- Get 10 units per month
- Download Article/Chapter or eBook
- 1 Unit = 1 Article or 1 Chapter
- Cancel anytime
- Available as PDF
- Read on any device
- Instant download
- Own it forever
- Available as EPUB and PDF
- Compact, lightweight edition
- Dispatched in 3 to 5 business days
- Free shipping worldwide - see info
- Durable hardcover edition
Tax calculation will be finalised at checkout
Purchases are for personal use only
Institutional subscriptions
Similar content being viewed by others
Design Thinking
Looking Inside the Box to Think Outside It: Contextualizing Design Thinking
Teaching with Design Thinking: Developing New Vision and Approaches to Twenty-First Century Learning
Author information, authors and affiliations.
Hasso Plattner Institute Academy, August-Bebel-Str. 88, 14482, Potsdam, Germany
Hasso Plattner Institute for Digital Engineering, Campus Griebnitzsee, Prof.-Dr.-Helmert-Str. 2-3, 14482, Potsdam, Germany
Christoph Meinel
You can also search for this author in PubMed Google Scholar
Editor information
Editors and affiliations.
Hasso Plattner Institute, Potsdam, Germany
HPI Academy, Hasso Plattner Institute, Potsdam, Germany
Rights and permissions
Reprints and permissions
Copyright information
© 2022 The Author(s), under exclusive license to Springer Nature Switzerland AG
About this chapter
Krohn, T., Meinel, C. (2022). Introduction: Design Thinking in the Field of Education. In: Meinel, C., Krohn, T. (eds) Design Thinking in Education. Springer, Cham. https://doi.org/10.1007/978-3-030-89113-8_1
Download citation
DOI : https://doi.org/10.1007/978-3-030-89113-8_1
Published : 13 April 2022
Publisher Name : Springer, Cham
Print ISBN : 978-3-030-89112-1
Online ISBN : 978-3-030-89113-8
eBook Packages : Education Education (R0)
Share this chapter
Anyone you share the following link with will be able to read this content:
Sorry, a shareable link is not currently available for this article.
Provided by the Springer Nature SharedIt content-sharing initiative
- Publish with us
Policies and ethics
- Find a journal
- Track your research
- Reviews / Why join our community?
- For companies
- Frequently asked questions
Design Thinking (DT)
What is design thinking (dt).
Design thinking is a non-linear, iterative process that teams use to understand users, challenge assumptions, redefine problems and create innovative solutions to prototype and test. It is most useful to tackle ill-defined or unknown problems and involves five phases: Empathize, Define, Ideate, Prototype and Test.
- Transcript loading…
Why Is Design Thinking so Important?
“Design thinking is a human-centered approach to innovation that draws from the designer's toolkit to integrate the needs of people, the possibilities of technology, and the requirements for business success.” — Tim Brown, CEO of IDEO
Design thinking fosters innovation . Companies must innovate to survive and remain competitive in a rapidly changing environment. In design thinking, cross-functional teams work together to understand user needs and create solutions that address those needs. Moreover, the design thinking process helps unearth creative solutions.
Design teams use design thinking to tackle ill-defined/unknown problems (aka wicked problems ). Alan Dix, Professor of Human-Computer Interaction, explains what wicked problems are in this video.
Wicked problems demand teams to think outside the box, take action immediately, and constantly iterate—all hallmarks of design thinking.
Don Norman, a pioneer of user experience design, explains why the designer’s way of thinking is so powerful when it comes to such complex problems.
Design thinking offers practical methods and tools that major companies like Google, Apple and Airbnb use to drive innovation. From architecture and engineering to technology and services, companies across industries have embraced the methodology to drive innovation and address complex problems.
The End Goal of Design Thinking: Be Desirable, Feasible and Viable
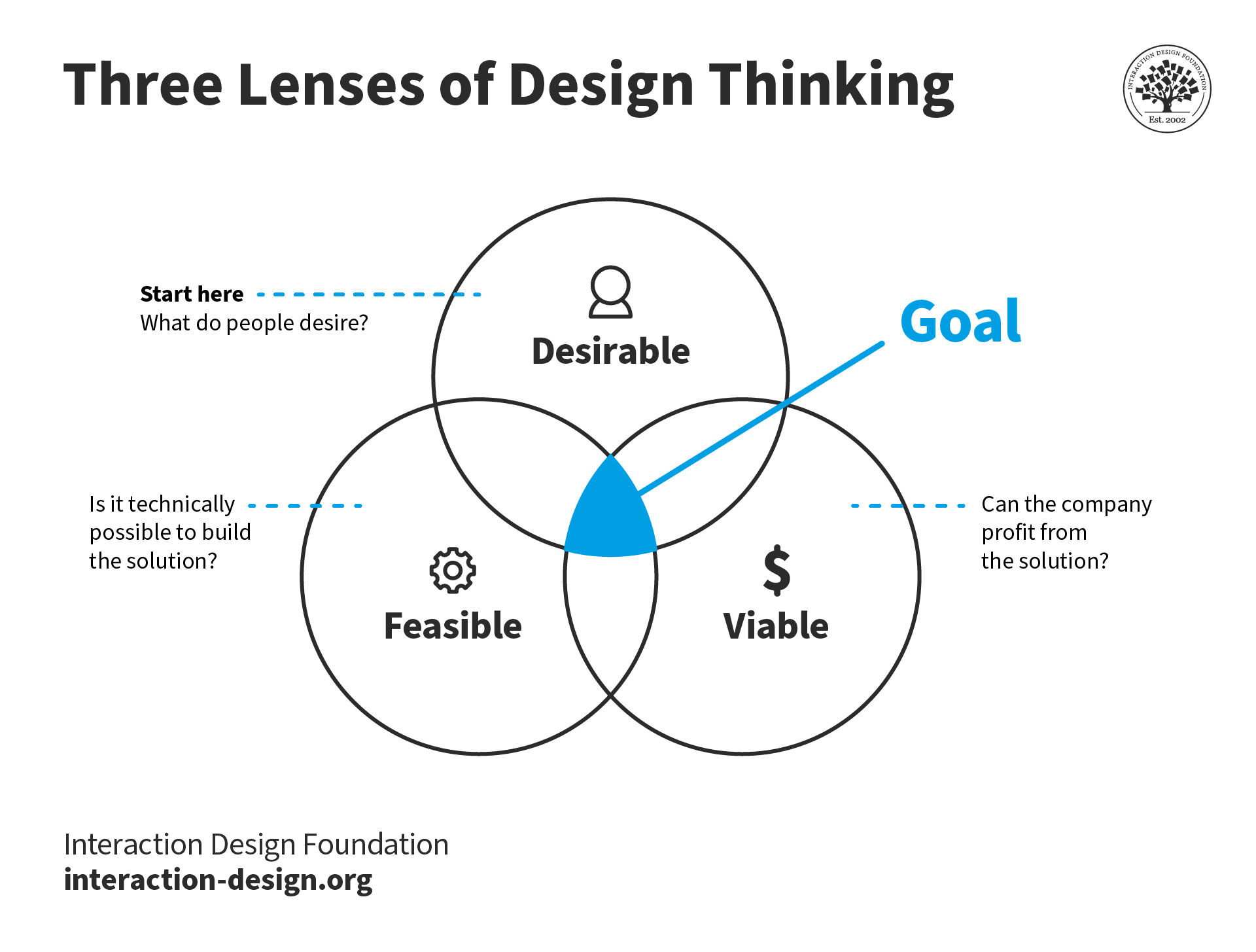
The design thinking process aims to satisfy three criteria: desirability (what do people desire?), feasibility (is it technically possible to build the solution?) and viability (can the company profit from the solution?). Teams begin with desirability and then bring in the other two lenses.
© Interaction Design Foundation, CC BY-SA 4.0
Desirability: Meet People’s Needs
The design thinking process starts by looking at the needs, dreams and behaviors of people—the end users. The team listens with empathy to understand what people want, not what the organization thinks they want or need. The team then thinks about solutions to satisfy these needs from the end user’s point of view.
Feasibility: Be Technologically Possible
Once the team identifies one or more solutions, they determine whether the organization can implement them. In theory, any solution is feasible if the organization has infinite resources and time to develop the solution. However, given the team’s current (or future resources), the team evaluates if the solution is worth pursuing. The team may iterate on the solution to make it more feasible or plan to increase its resources (say, hire more people or acquire specialized machinery).
At the beginning of the design thinking process, teams should not get too caught up in the technical implementation. If teams begin with technical constraints, they might restrict innovation.

Viability: Generate Profits
A desirable and technically feasible product isn’t enough. The organization must be able to generate revenues and profits from the solution. The viability lens is essential not only for commercial organizations but also for non-profits.
Traditionally, companies begin with feasibility or viability and then try to find a problem to fit the solution and push it to the market. Design thinking reverses this process and advocates that teams begin with desirability and bring in the other two lenses later.
The Five Stages of Design Thinking
Stanford University’s Hasso Plattner Institute of Design, commonly known as the d.school, is renowned for its pioneering approach to design thinking. Their design process has five phases: Empathize, Define, Ideate, Prototype, and Test. These stages are not always sequential. Teams often run them in parallel, out of order, and repeat them as needed.
Stage 1: Empathize —Research Users' Needs
The team aims to understand the problem, typically through user research. Empathy is crucial to design thinking because it allows designers to set aside your assumptions about the world and gain insight into users and their needs.
Stage 2: Define—State Users' Needs and Problems
Once the team accumulates the information, they analyze the observations and synthesize them to define the core problems. These definitions are called problem statements . The team may create personas to help keep efforts human-centered.
Stage 3: Ideate—Challenge Assumptions and Create Ideas
With the foundation ready, teams gear up to “think outside the box.” They brainstorm alternative ways to view the problem and identify innovative solutions to the problem statement.
Stage 4: Prototype—Start to Create Solutions
This is an experimental phase. The aim is to identify the best possible solution for each problem. The team produces inexpensive, scaled-down versions of the product (or specific features found within the product) to investigate the ideas. This may be as simple as paper prototypes .
Stage 5: Test—Try the Solutions Out
The team tests these prototypes with real users to evaluate if they solve the problem. The test might throw up new insights, based on which the team might refine the prototype or even go back to the Define stage to revisit the problem.
These stages are different modes that contribute to the entire design project rather than sequential steps. The goal is to gain a deep understanding of the users and their ideal solution/product.
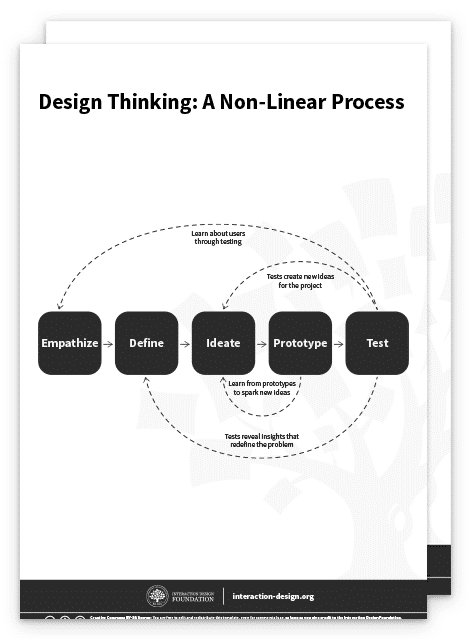
Design Thinking Frameworks
There is no single definition or process for design thinking. The five-stage design thinking methodology described above is just one of several frameworks.
Hasso-Platner Institute Panorama
Ludwig Wilhelm Wall, CC BY-SA 3.0 , via Wikimedia Commons
Innovation doesn’t follow a linear path or have a clear-cut formula. Global design leaders and consultants have interpreted the abstract design process in different ways and have proposed other frameworks of design thinking.
Head, Heart and Hand by the American Institution of Graphic Arts (AIGA)
The Head, Heart, and Hand approach by AIGA (American Institute of Graphic Arts) is a holistic perspective on design. It integrates the intellectual, emotional, and practical aspects of the creative process.
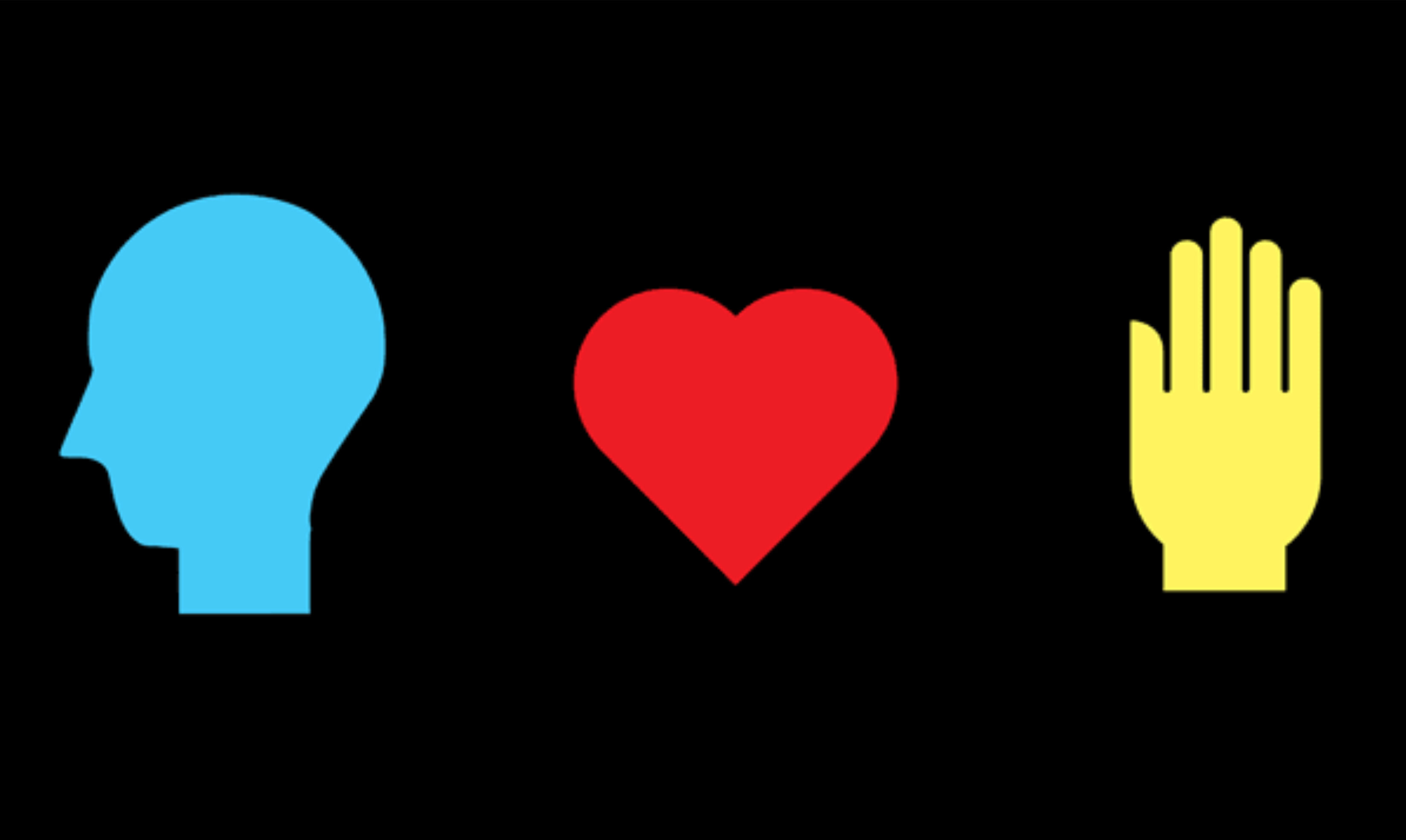
More than a process, the Head, Heart and Hand framework outlines the different roles that designers must perform to create great results.
© American Institute of Graphic Arts, Fair Use
“ Head ” symbolizes the intellectual component. The team focuses on strategic thinking, problem-solving and the cognitive aspects of design. It involves research and analytical thinking to ensure that design decisions are purposeful.
“ Heart ” represents the emotional dimension. It emphasizes empathy, passion, and human-centeredness. This aspect is crucial in understanding the users’ needs, desires, and experiences to ensure that designs resonate on a deeper, more personal level.
“ Hand ” signifies the practical execution of ideas, the craftsmanship, and the skills necessary to turn concepts into tangible solutions. This includes the mastery of tools, techniques, and materials, as well as the ability to implement and execute design ideas effectively.
Inspire, Ideate, Implement by IDEO
IDEO is a leading design consultancy and has developed its own version of the design thinking framework.
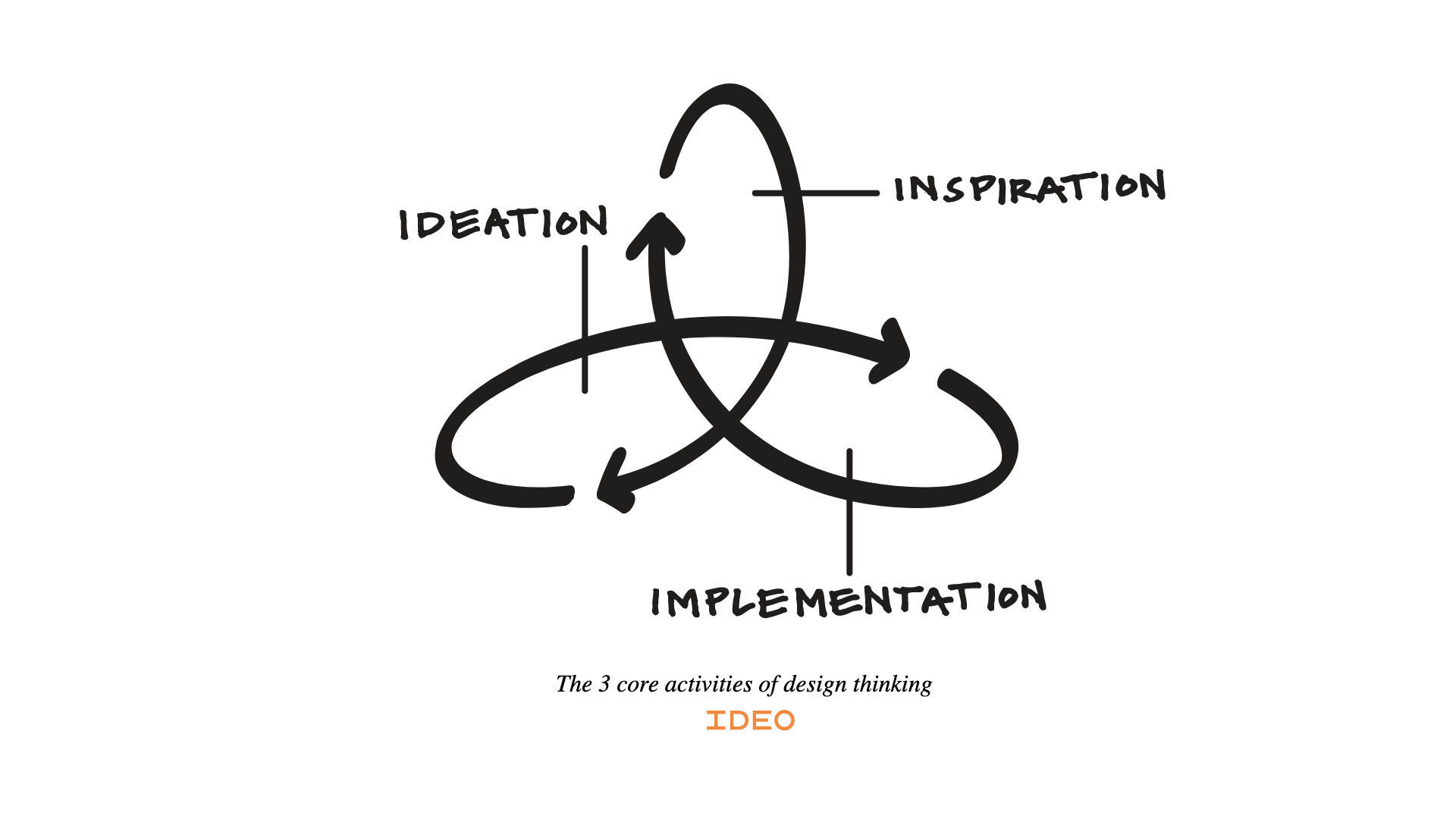
IDEO’s design thinking process is a cyclical three-step process that involves Inspiration, Ideation and Implementation.
© IDEO, Public License
In the “ Inspire ” phase, the team focuses on understanding users’ needs, behaviors, and motivations. The team empathizes with people through observation and user interviews to gather deep insights.
In the “ Ideate ” phase, the team synthesizes the insights gained to brainstorm a wide array of creative solutions. This stage encourages divergent thinking, where teams focus on quantity and variety of ideas over immediate practicality. The goal is to explore as many possibilities as possible without constraints.
In the “ Implement ” phase, the team brings these ideas to life through prototypes. The team tests, iterates and refines these ideas based on user feedback. This stage is crucial for translating abstract concepts into tangible, viable products, services, or experiences.
The methodology emphasizes collaboration and a multidisciplinary approach throughout each phase to ensure solutions are innovative and deeply rooted in real human needs and contexts.
The Double Diamond by the Design Council
In the book Designing Social Systems in a Changing World , Béla Heinrich Bánáthy, Professor at San Jose State University and UC Berkeley, created a “divergence-convergence model” diagram. The British Design Council interpreted this diagram to create the Double Diamond design process model.
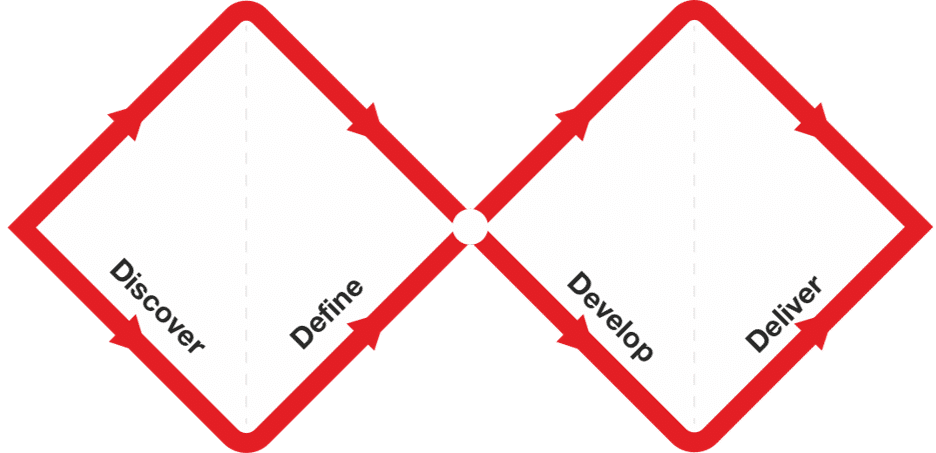
As the name suggests, the double diamond model consists of two diamonds—one for the problem space and the other for the solution space. The model uses diamonds to represent the alternating diverging and converging activities.
© Design Council, CC BY 4.0
In the diverging “ Discover ” phase, designers gather insights and empathize with users’ needs. The team then converges in the “ Define ” phase to identify the problem.
The second, solution-related diamond, begins with “ Develop ,” where the team brainstorms ideas. The final stage is “ Deliver ,” where the team tests the concepts and implements the most viable solution.
This model balances expansive thinking with focused execution to ensure that design solutions are both creative and practical. It underscores the importance of understanding the problem thoroughly and carefully crafting the solution, making it a staple in many design and innovation processes.
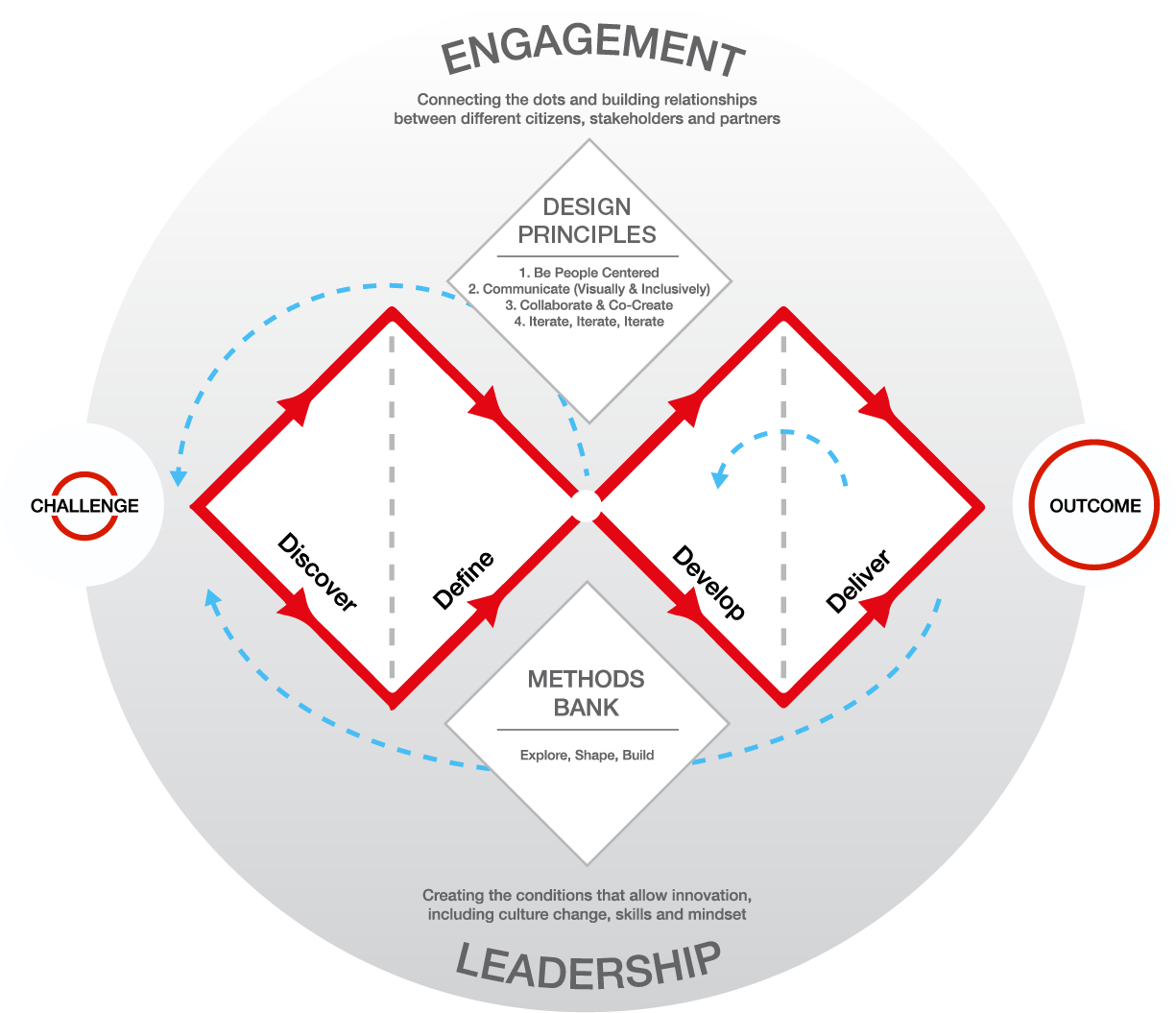
With the widespread adoption of the double diamond framework, Design Council’s simple visual evolved.
In this expanded and annotated version, the framework emphasizes four design principles:
Be people-centered.
Communicate (visually and inclusively).
Collaborate and co-create.
Iterate, iterate, iterate!
The updated version also highlights the importance of leadership (to create an environment that allows innovation) and engagement (to connect with different stakeholders and involve them in the design process).
Common Elements of Design Thinking Frameworks
On the surface, design thinking frameworks look very different—they use alternative names and have different numbers of steps. However, at a fundamental level, they share several common traits.
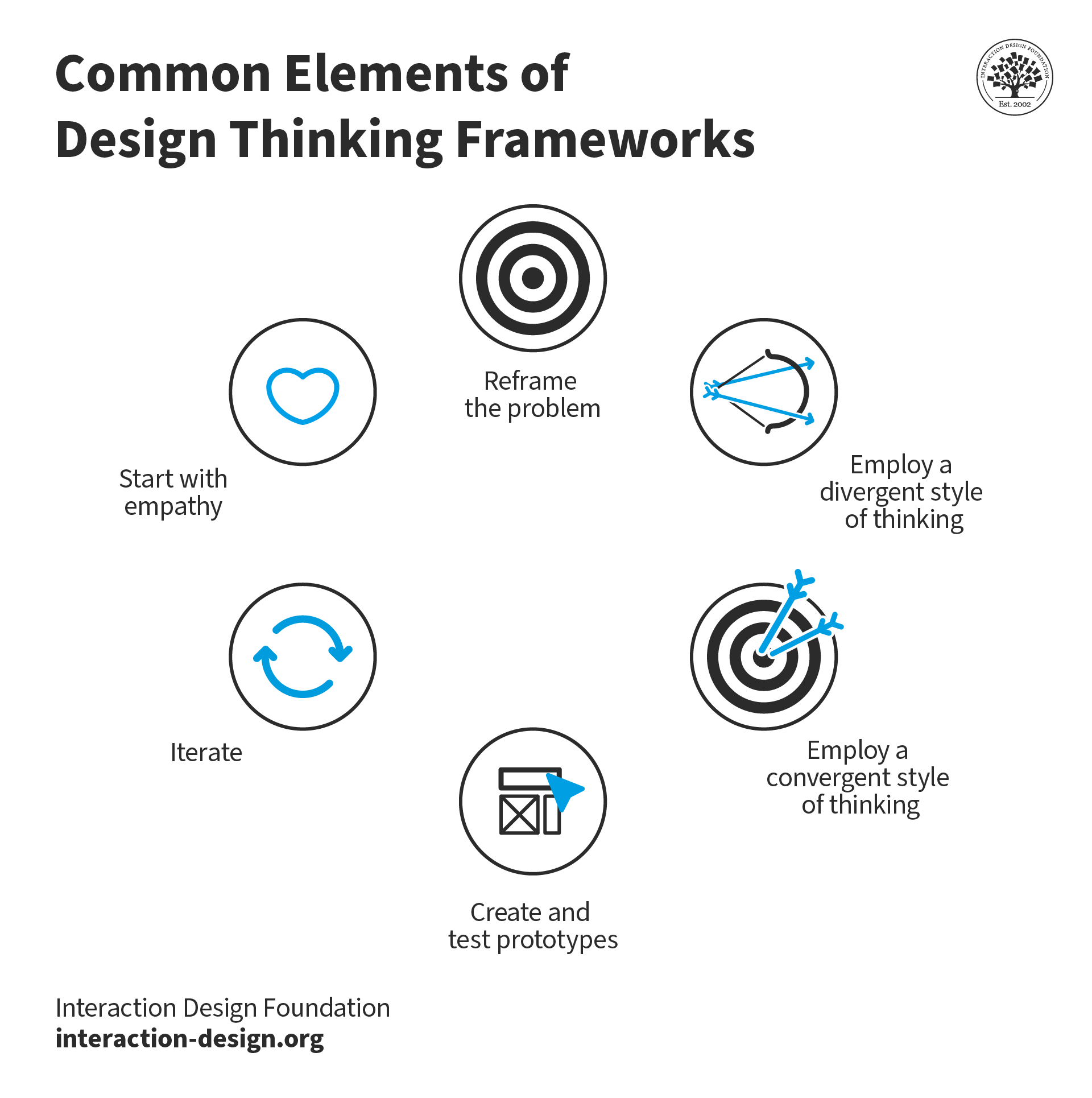
Start with empathy . Focus on the people to come up with solutions that work best for individuals, business, and society.
Reframe the problem or challenge at hand . Don’t rush into a solution. Explore the problem space and look at the issue through multiple perspectives to gain a more holistic, nuanced understanding.
Initially, employ a divergent style of thinking (analyze) . In the problem space, gather as many insights as possible. In the solution space, encourage team members to generate and explore as many solutions as possible in an open, judgment-free ideation space.
Later, employ a convergent style of thinking (synthesize) . In the problem space, synthesize all data points to define the problem. In the solution space, whittle down all the ideas—isolate, combine and refine potential solutions to create more mature ideas.
Create and test prototypes . Solutions that make it through the previous stages get tested further to remove potential issues.
Iterate . As the team progresses through the various stages, they revisit different stages and may redefine the challenge based on new insights.
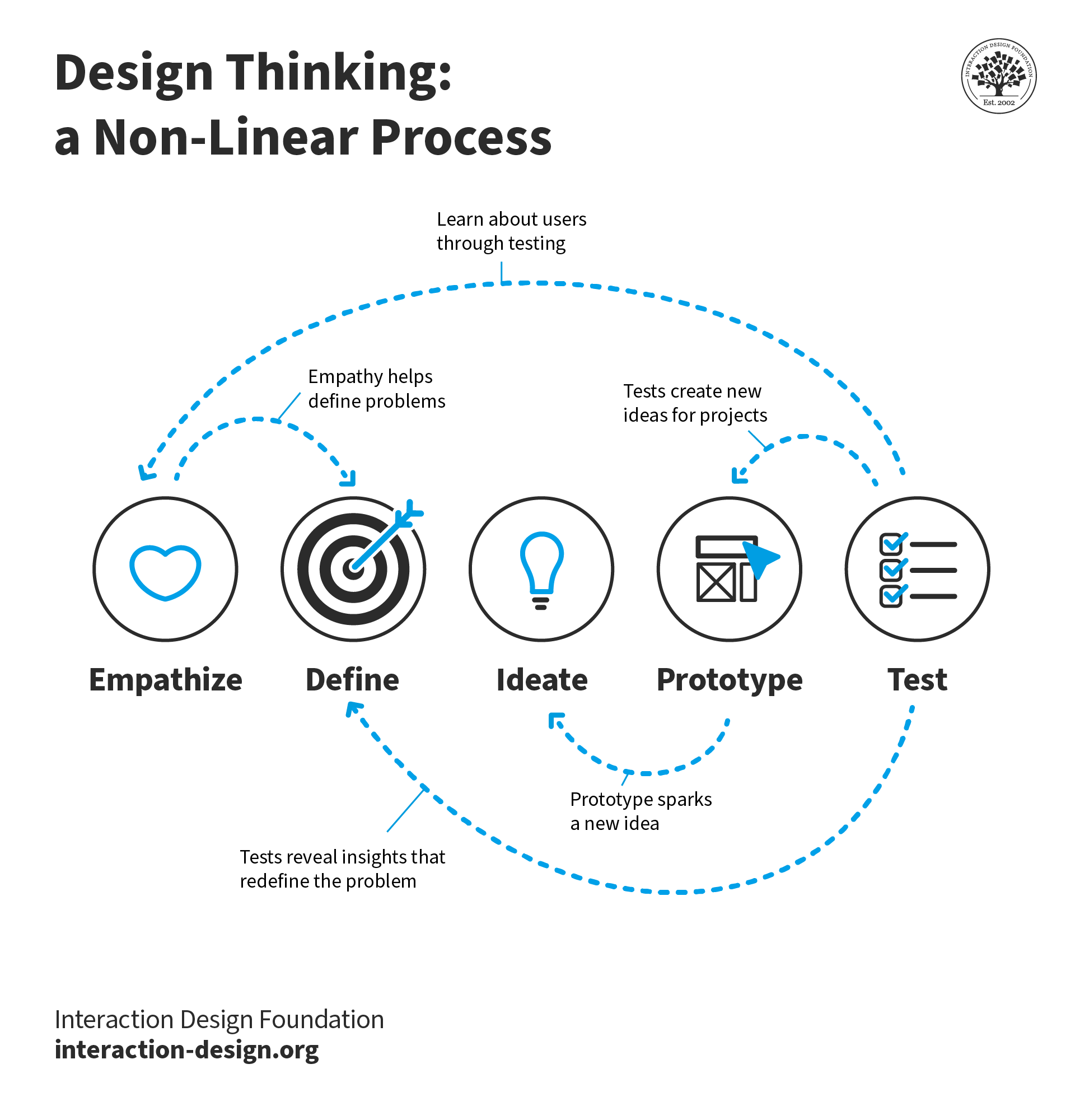
Design thinking is a non-linear process. For example, teams may jump from the test stage to the define stage if the tests reveal insights that redefine the problem. Or, a prototype might spark a new idea, prompting the team to step back into the ideate stage. Tests may also create new ideas for projects or reveal insights about users.
Design Thinking Mindsets: More than a Process
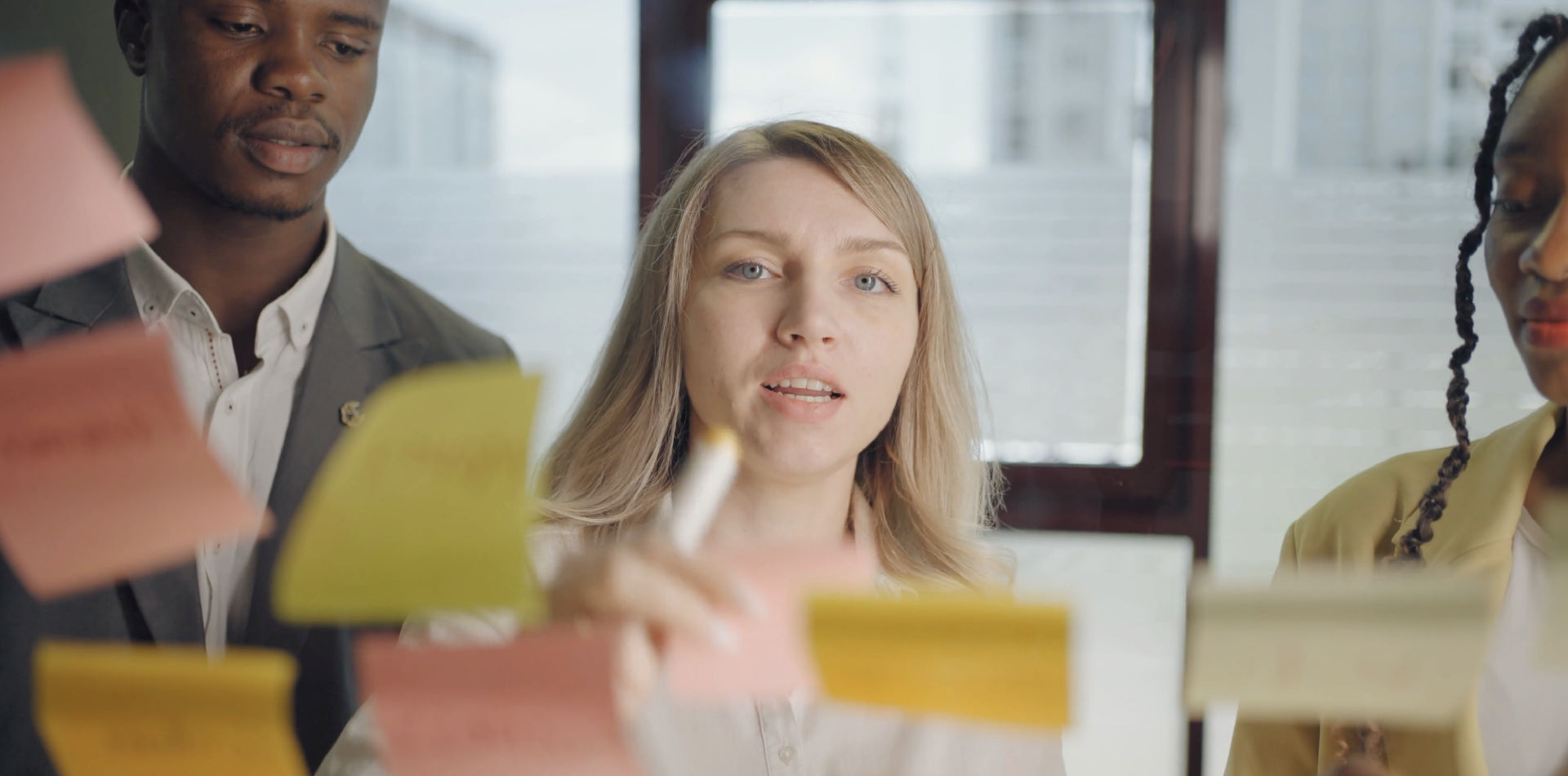
A mindset is a characteristic mental attitude that determines how one interprets and responds to situations . Design thinking mindsets are how individuals think , feel and express themselves during design thinking activities. It includes people’s expectations and orientations during a design project.
Without the right mindset, it can be very challenging to change how we work and think.
The key mindsets that ensure a team can successfully implement design thinking are.
Be empathetic: Empathy is the ability to place yourself, your thinking and feelings in another person’s shoes. Design thinking begins from a deep understanding of the needs and motivations of people—the parents, neighbors, children, colleagues, and strangers who make up a community.
Be collaborative: No one person is responsible for the outcome when you work in a team. Several great minds are always stronger than just one. Design thinking benefits from the views of multiple perspectives and lets others’ creativity bolster your own.
Be optimistic: Be confident about achieving favorable outcomes. Design thinking is the fundamental belief that we can all create change—no matter how big a problem, how little time, or how small a budget. Designing can be a powerful process no matter what constraints exist around you.
Embrace ambiguity: Get comfortable with ambiguous and complex situations. If you expect perfection, it is difficult to take risks, which limits your ability to create radical change. Design thinking is all about experimenting and learning by doing. It gives you the confidence to believe that new, better things are possible and that you can help make them a reality.
Be curious: Be open to different ideas. Recognize that you are not the user.
Reframe: Challenge and reframe assumptions associated with a given situation or problem. Don’t take problems at face value. Humans are primed to look for patterns. The unfortunate side effect of these patterns is that we form (often false and sometimes dangerous) stereotypes and assumptions. Design thinking aims to help you break through any preconceived notions and biases and reframe challenges.
Embrace diversity: Work with and engage people with different cultural backgrounds, experiences, and ways of thinking and working. Everyone brings a unique perspective to the team. When you include diverse voices in a team, you learn from each other’s experiences, further helping you break through your assumptions.
Make tangible: When you make ideas tangible, it is faster and easier for everyone on the team to be on the same page. For example, sketching an idea or enacting a scenario is far more convenient and easy to interpret than an elaborate presentation or document.
Take action: Run experiments and learn from them.
Design Thinking vs Agile Methodology
Teams often use design thinking and agile methodologies in project management, product development, and software development. These methodologies have distinct approaches but share some common principles.
Similarities between Design Thinking and Agile
Iterative process.
Both methodologies emphasize iterative development. In design thinking, teams may jump from one phase to another, not necessarily in a set cyclical or linear order. For example, on testing a prototype, teams may discover something new about their users and realize that they must redefine the problem. Agile teams iterate through development sprints.
User-Centered
The agile and design thinking methodologies focus on the end user. All design thinking activities—from empathizing to prototyping and testing—keep the end users front and center. Agile teams continually integrate user feedback into development cycles.
Collaboration and Teamwork
Both methodologies rely heavily on collaboration among cross-functional teams and encourage diverse perspectives and expertise.
Flexibility and Adaptability
With its focus on user research, prototyping and testing, design thinking ensures teams remain in touch with users and get continuous feedback. Similarly, agile teams monitor user feedback and refine the product in a reasonably quick time.
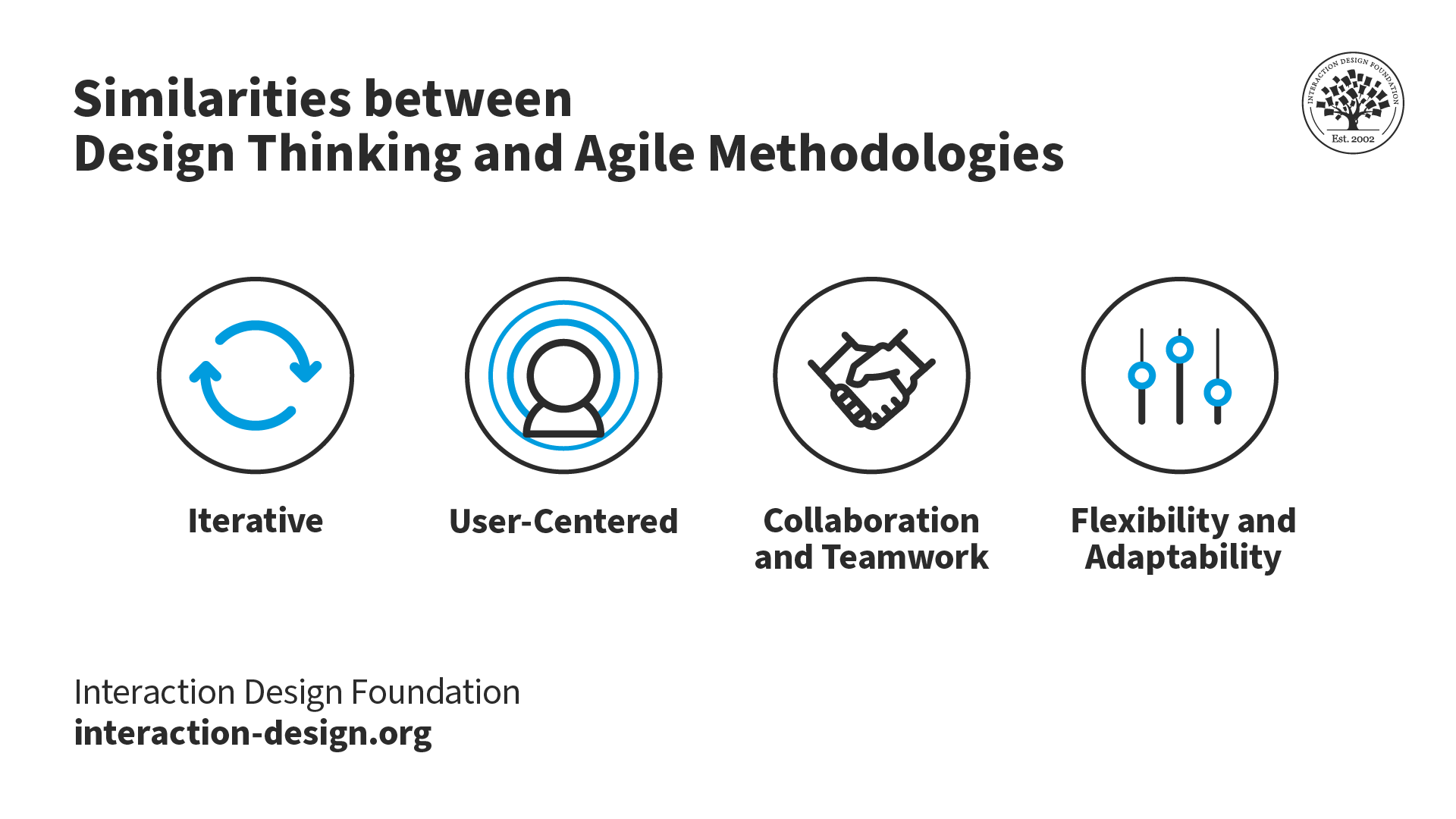
In this video, Laura Klein, author of Build Better Products , describes a typical challenge designers face on agile teams. She encourages designers to get comfortable with the idea of a design not being perfect. Notice the many parallels between Laura’s advice for designers on agile teams and the mindsets of design thinking.
Differences between Design Thinking and Agile
While design thinking and agile teams share principles like iteration, user focus, and collaboration, they are neither interchangeable nor mutually exclusive. A team can apply both methodologies without any conflict.
From a user experience design perspective, design thinking applies to the more abstract elements of strategy and scope. At the same time, agile is more relevant to the more concrete elements of UX: structure, skeleton and surface. For quick reference, here’s an overview of the five elements of user experience.
Design thinking is more about exploring and defining the right problem and solution, whereas agile is about efficiently executing and delivering a product.
Here are the key differences between design thinking and agile.
|
|
|
| It primarily originates in design and borrows from multiple disciplines, including psychology, systems thinking, and business strategy. | It primarily originates from software development and borrows from disciplines such as manufacturing and project management. |
| Problem-solving and innovative solutions. | Efficient product delivery. |
| Usually, toward the beginning of a project. Aims to define the problem and test and pick a solution. | Usually, after teams have a clear solution. Aims to deliver that solution and continuously iterate on the live product. |
| Fluid process, less formal and relatively lesser documentation. | Structured and formal process with extensive documentation. |
| An idea or solution, usually with a prototype, may not be tangible. | Tangible, working product (usually software) shipped to end users. |
Design Sprint: A Condensed Version of Design Thinking
A design sprint is a 5-day intensive workshop where cross-functional teams aim to develop innovative solutions.
The design sprint is a very structured version of design thinking that fits into the timeline of a sprint (a sprint is a short timeframe in which agile teams work to produce deliverables). Developed by Google Ventures, the design sprint seeks to fast-track innovation.
In this video, user researcher Ditte Hvas Mortensen explains the design sprint in detail.
Learn More about Design Thinking
Design consultancy IDEO’s designkit is an excellent repository of design thinking tools and case studies.
To keep up with recent developments in design thinking, read IDEO CEO Tim Brown’s blog .
Enroll in our course Design Thinking: The Ultimate Guide —an excellent guide to get you started on your design thinking projects.
Questions related to Design Thinking
You don’t need any certification to practice design thinking. However, learning about the nuances of the methodology can help you:
Pick the appropriate methods and tailor the process to suit the unique needs of your project.
Avoid common pitfalls when you apply the methods.
Better lead a team and facilitate workshops.
Increase the chances of coming up with innovative solutions.
IxDF has a comprehensive course to help you gain the most from the methodology: Design Thinking: The Ultimate Guide .
Anyone can apply design thinking to solve problems. Despite what the name suggests, non-designers can use the methodology in non-design-related scenarios. The methodology helps you think about problems from the end user’s perspective. Some areas where you can apply this process:
Develop new products with greater chances of success.
Address community-related issues (such as education, healthcare and environment) to improve society and living standards.
Innovate/enhance existing products to gain an advantage over the competition.
Achieve greater efficiencies in operations and reduce costs.
Use the Design Thinking: The Ultimate Guide course to apply design thinking to your context today.
A framework is the basic structure underlying a system, concept, or text. There are several design thinking frameworks with slight differences. However, all the frameworks share some traits. Each framework:
Begins with empathy.
Reframes the problem or challenge at hand.
Initially employs divergent styles of thinking to generate ideas.
Later, it employs convergent styles of thinking to narrow down the best ideas,
Creates and tests prototypes.
Iterates based on the tests.
Some of the design thinking frameworks are:
5-stage design process by d.school
7-step early traditional design process by Herbert Simon
The 5-Stage DeepDive™ by IDEO
The “Double Diamond” Design Process Model by the Design Council
Collective Action Toolkit (CAT) by Frog Design
The LUMA System of Innovation by LUMA Institute
For details about each of these frameworks, see 10 Insightful Design Thinking Frameworks: A Quick Overview .
IDEO’s 3-Stage Design Thinking Process consists of inspiration, ideation and implementation:
Inspire : The problem or opportunity inspires and motivates the search for a solution.
Ideate : A process of synthesis distills insights which can lead to solutions or opportunities for change.
Implement : The best ideas are turned into a concrete, fully conceived action plan.
IDEO is a leader in applying design thinking and has developed many frameworks. Find out more in 10 Insightful Design Thinking Frameworks: A Quick Overview .
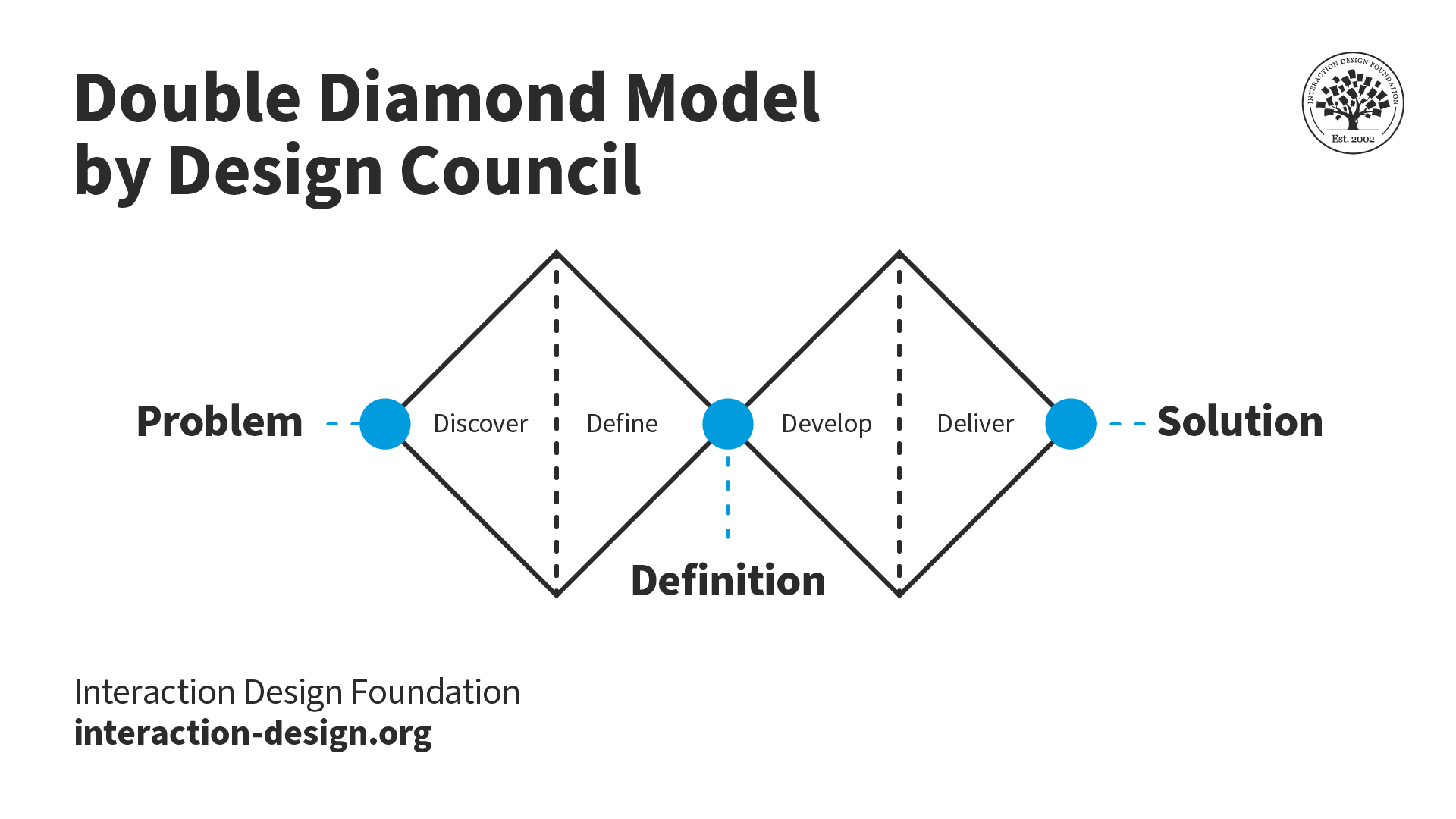
Design Council's Double Diamond diagram depicts the divergent and convergent stages of the design process.
Béla H. Bánáthy, founder of the White Stag Leadership Development Program, created the “divergence-convergence” model in 1996. In the mid-2000s, the British Design Council made this famous as the Double Diamond model.
The Double Diamond diagram graphically represents a design thinking process. It highlights the divergent and convergent styles of thinking in the design process. It has four distinct phases:
Discover: Initial idea or inspiration based on user needs.
Define: Interpret user needs and align them with business objectives.
Develop: Develop, iterate and test design-led solutions.
Deliver: Finalize and launch the end product into the market.
Double Diamond is one of several design thinking frameworks. Find out more in 10 Insightful Design Thinking Frameworks: A Quick Overview .
There are several design thinking methods that you can choose from, depending on what stage of the process you’re in. Here are a few common design thinking methods:
User Interviews: to understand user needs, pain points, attitudes and behaviors.
5 Whys Method: to dig deeper into problems to diagnose the root cause.
User Observations: to understand how users behave in real life (as opposed to what they say they do).
Affinity Diagramming: to organize research findings.
Empathy Mapping: to empathize with users based on research insights.
Journey Mapping: to visualize a user’s experience as they solve a problem.
6 Thinking Hats: to encourage a group to think about a problem or solution from multiple perspectives.
Brainstorming: to generate ideas.
Prototyping: to make abstract ideas more tangible and test them.
Dot Voting: to select ideas.
Start applying these methods to your work today with the Design Thinking template bundle .
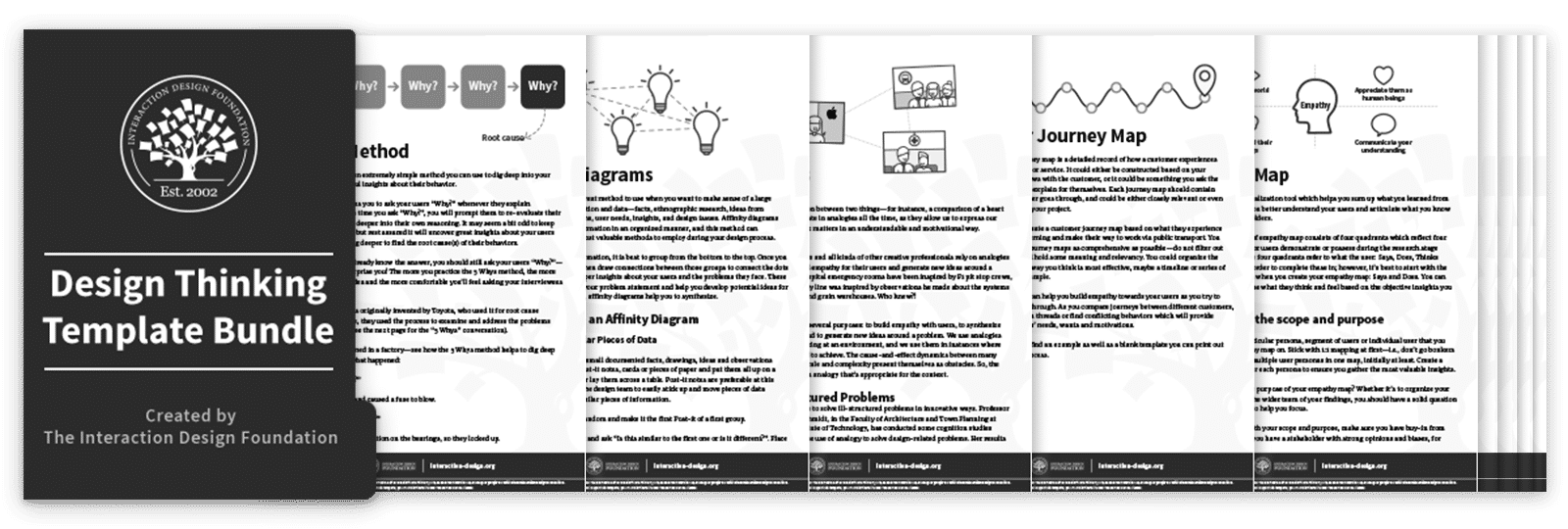
For most of the design thinking process, you will need basic office stationery:
Pen and paper
Sticky notes
Whiteboard and markers
Print-outs of templates and canvases as needed (such as empathy maps, journey maps, feedback capture grid etc.) You can also draw these out manually.
Prototyping materials such as UI stencils, string, clay, Lego bricks, sticky tapes, scissors and glue.
A space to work in.
You can conduct design thinking workshops remotely by:
Using collaborative software to simulate the whiteboard and sticky notes.
Using digital templates instead of printed canvases.
Download print-ready templates you can share with your team to practice design thinking today.
Design thinking is a problem-solving methodology that helps teams better identify, understand, and solve business and customer problems.
When businesses prioritize and empathize with customers, they can create solutions catering to their needs. Happier customers are more likely to be loyal and organically advocate for the product.
Design thinking helps businesses develop innovative solutions that give them a competitive advantage.
Gain a competitive advantage in your business with Design Thinking: The Ultimate Guide .
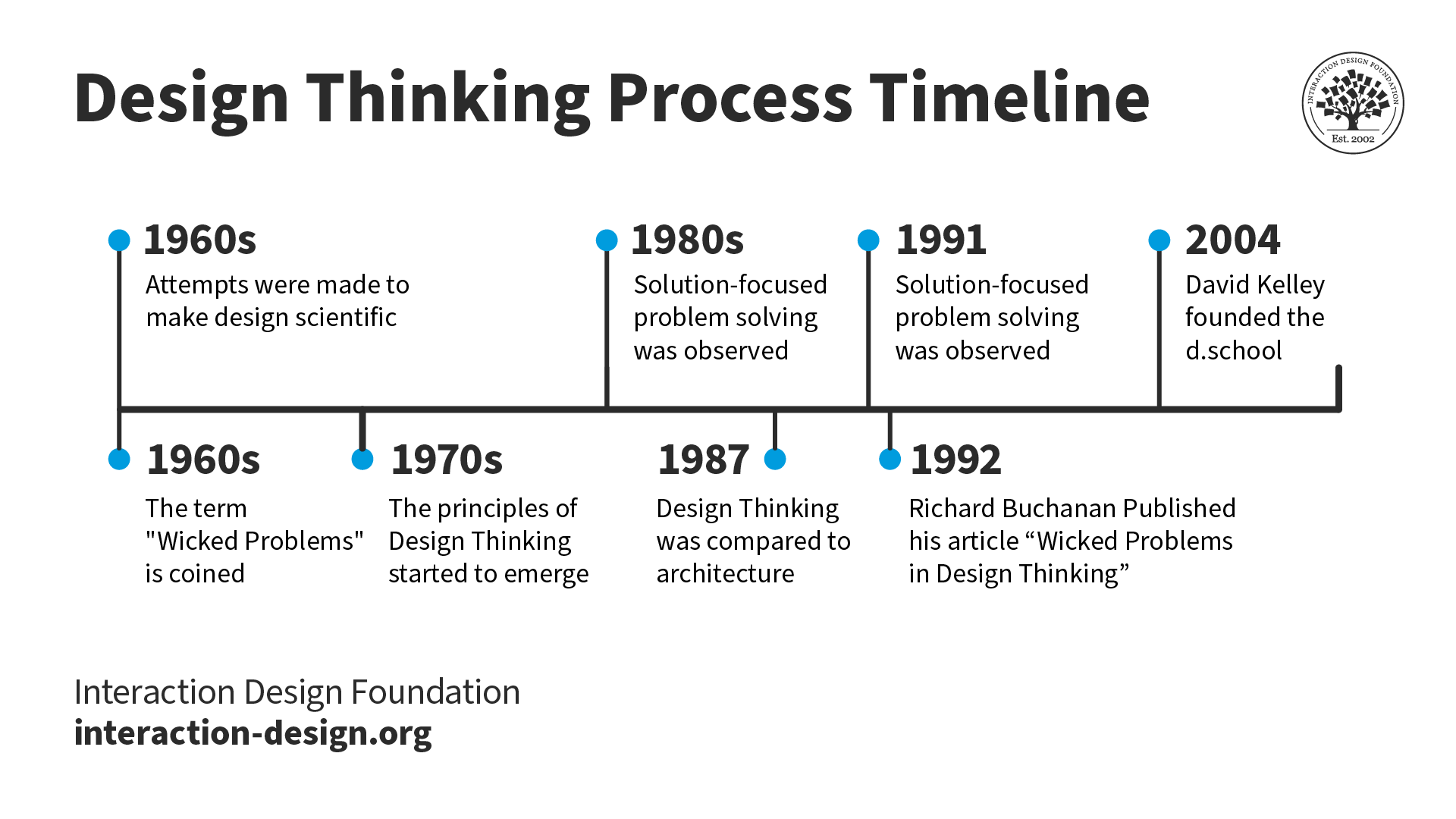
The evolution of Design Thinking can be summarised in 8 key events from the 1960s to 2004.
© Interaction Design Foundation, CC BY-SA 4.0.
Herbert Simon’s 1969 book, "The Sciences of the Artificial," has one of the earliest references to design thinking. David Kelley, founder of the design consultancy IDEO, coined the term “design thinking” and helped make it popular.
For a more comprehensive discussion on the origins of design thinking, see The History of Design Thinking .
Some organizations that have employed design thinking successfully are:
Airbnb: Airbnb used design thinking to create a platform for people to rent out their homes to travelers. The company focused on the needs of both hosts and guests . The result was a user-friendly platform to help people find and book accommodations.
PillPack: PillPack is a prescription home-delivery system. The company focused on the needs of people who take multiple medications and created a system that organizes pills by date and time. Amazon bought PillPack in 2018 for $1 billion .
Google Creative Lab: Google Creative Lab collaborated with IDEO to discover how kids physically play and learn. The team used design thinking to create Project Bloks . The project helps children develop foundational problem-solving skills "through coding experiences that are playful, tactile and collaborative.”
See more examples of design thinking and learn practical methods in Design Thinking: The Ultimate Guide .
Innovation essentially means a new idea. Design thinking is a problem-solving methodology that helps teams develop new ideas. In other words, design thinking can lead to innovation.
Human-Centered Design is a newer term for User-Centered Design
“Human-centred design is an approach to interactive systems development that aims to make systems usable and useful by focusing on the users, their needs and requirements, and by applying human factors/ergonomics, and usability knowledge and techniques. This approach enhances effectiveness and efficiency, improves human well-being, user satisfaction, accessibility and sustainability; and counteracts possible adverse effects of use on human health, safety and performance.”
— ISO 9241-210:2019(en), ISO (the International Organization for Standardization)
User experience expert Don Norman describes human-centered design (HCD) as a more evolved form of user-centered design (UCD). The word "users" removes their importance and treats them more like objects than people. By replacing “user” with “human,” designers can empathize better with the people for whom they are designing. Don Norman takes HCD a step further and prefers the term People-Centered Design.
Design thinking has a broader scope and takes HCD beyond the design discipline to drive innovation.
People sometimes use design thinking and human-centered design to mean the same thing. However, they are not the same. HCD is a formal discipline with a specific process used only by designers and usability engineers to design products. Design thinking borrows the design methods and applies them to problems in general.
Design Sprint condenses design thinking into a 1-week structured workshop
Google Ventures condensed the design thinking framework into a time-constrained 5-day workshop format called the Design Sprint. The sprint follows one step per day of the week:
Monday: Unpack
Tuesday: Sketch
Wednesday: Decide
Thursday: Prototype
Friday: Test
Learn more about the design sprint in Make Your UX Design Process Agile Using Google’s Methodology .
Systems Thinking is a distinct discipline with a broader approach to problem-solving
“Systems thinking is a way of exploring and developing effective action by looking at connected wholes rather than separate parts.”
— Introduction to Systems thinking, Report of GSE and GORS seminar, Civil Service Live
Both HCD and Systems Thinking are formal disciplines. Designers and usability engineers primarily use HCD. Systems thinking has applications in various fields, such as medical, environmental, political, economic, human resources, and educational systems.
HCD has a much narrower focus and aims to create and improve products. Systems thinking looks at the larger picture and aims to change entire systems.
Don Norman encourages designers to incorporate systems thinking in their work. Instead of looking at people and problems in isolation, designers must look at them from a systems point of view.
In summary, UCD and HCD refer to the same field, with the latter being a preferred phrase.
Design thinking is a broader framework that borrows methods from human-centered design to approach problems beyond the design discipline. It encourages people with different backgrounds and expertise to work together and apply the designer’s way of thinking to generate innovative solutions to problems.
Systems thinking is another approach to problem-solving that looks at the big picture instead of specific problems in isolation.
The design sprint is Google Ventures’ version of the design thinking process, structured to fit the design process in 1 week.
There are multiple design thinking frameworks, each with a different number of steps and phase names. One of the most popular frameworks is the Stanford d.School 5-stage process.
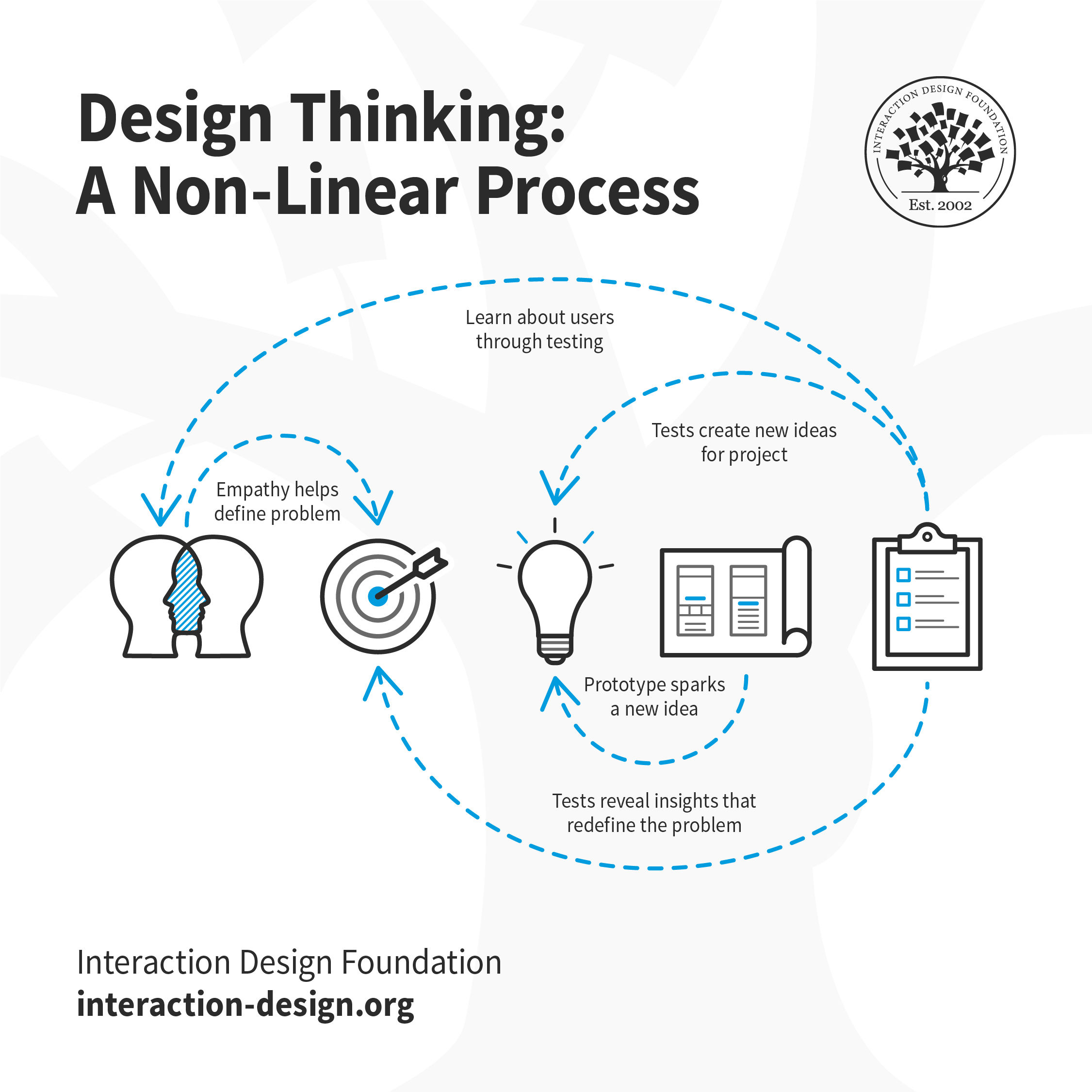
Design thinking is an iterative and non-linear process. It contains five phases: 1. Empathize, 2. Define, 3. Ideate, 4. Prototype and 5. Test. It is important to note the five stages of design thinking are not always sequential. They do not have to follow a specific order, and they can often occur in parallel or be repeated iteratively. The stages should be understood as different modes which contribute to the entire design project, rather than sequential steps.
For more details, see The 5 Stages in the Design Thinking Process .
IDEO is a leading design consultancy and has developed its own version of the design thinking framework and adds the dimension of implementation in the process.
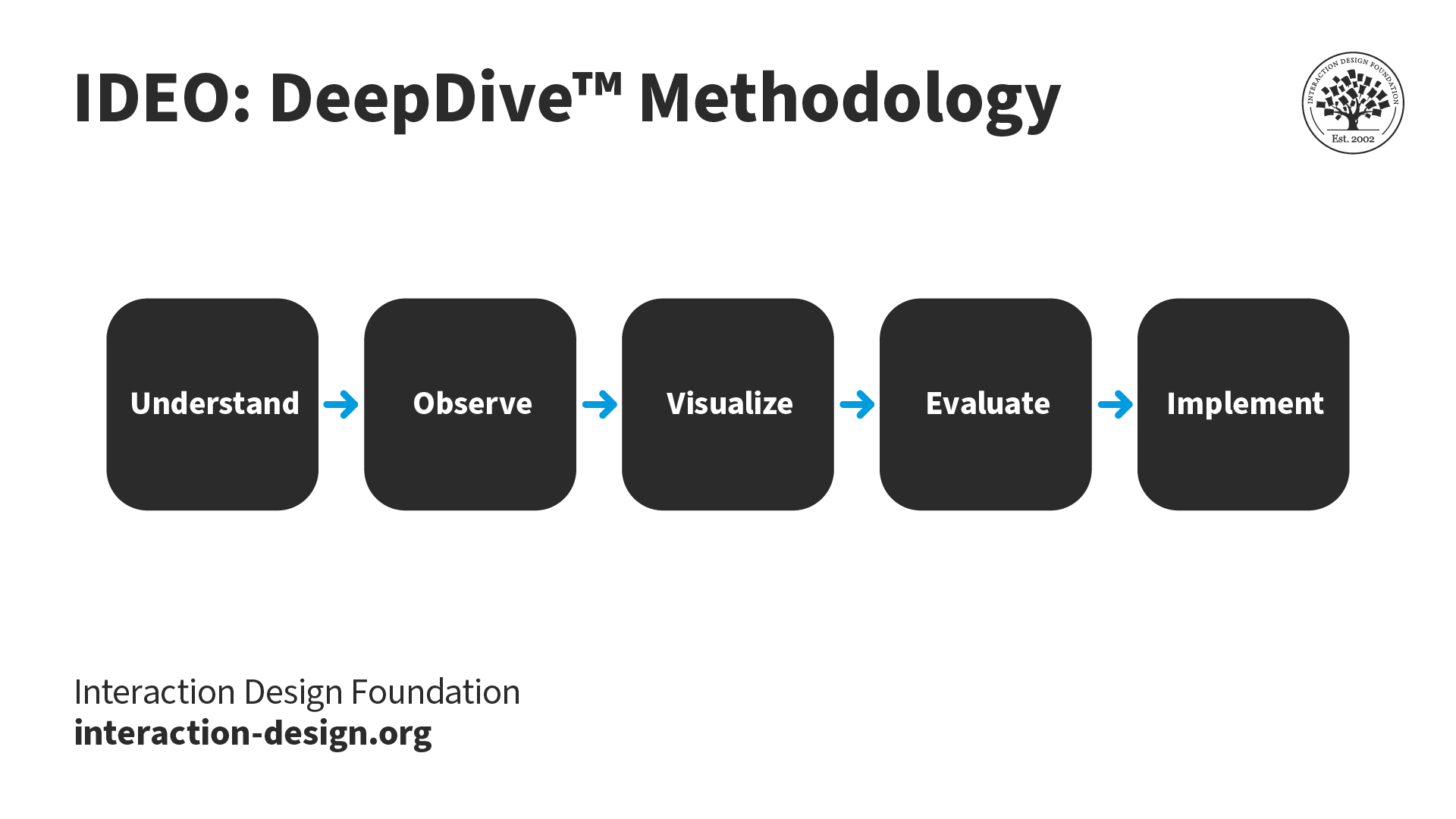
IDEO’s framework uses slightly different terms than d.school’s design thinking process and adds an extra dimension of implementation. The steps in the DeepDive™ Methodology are: Understand, Observe, Visualize, Evaluate and Implement.
IDEO’s DeepDive™ Methodology includes the following steps:
Understand: Conduct research and identify what the client needs and the market landscape
Observe: Similar to the Empathize step, teams observe people in live scenarios and conduct user research to identify their needs and pain points.
Visualize: In this step, the team visualizes new concepts. Similar to the Ideate phase, teams focus on creative, out-of-the-box and novel ideas.
Evaluate: The team prototypes ideas and evaluates them. After refining the prototypes, the team picks the most suitable one.
Implement: The team then sets about to develop the new concept for commercial use.
IDEO’s DeepDive™ is one of several design thinking frameworks. Find out more in 10 Insightful Design Thinking Frameworks: A Quick Overview .
Answer a Short Quiz to Earn a Gift
What are the stages in the design thinking process?
- Brainstorm, Prototype, Design, Launch, Test
- Define, Ideate, Research, Design, Test
- Empathize, Define, Ideate, Prototype, Test
Why is empathy critical in the design thinking process?
- It allows designers to understand and address the real needs of users.
- It helps designers maintain control over the creative process.
- It makes sure the solution is inexpensive and easy to create.
What is the primary purpose of the prototyping phase in design thinking?
- To explore potential solutions and how they might work in real-world situations
- To finalize the product design for mass production
- To sell the idea to stakeholders with a high-fidelity (hi-fi) demonstration
What is a "wicked problem" in design thinking?
- Problems that are complex, ill-defined and have no single correct answer.
- Problems that are straightforward and have a clear, single solution.
- Problems that are tricky, but can be solved quickly with conventional methods.
Why is the iterative process important in design thinking?
- It allows design teams to use up all available resources.
- It allows for the improvement of solutions based on user feedback and testing.
- It makes sure the solution remains unchanged throughout development.
Better luck next time!
Do you want to improve your UX / UI Design skills? Join us now
Congratulations! You did amazing
You earned your gift with a perfect score! Let us send it to you.
Check Your Inbox
We’ve emailed your gift to [email protected] .
Literature on Design Thinking (DT)
Here’s the entire UX literature on Design Thinking (DT) by the Interaction Design Foundation, collated in one place:
Learn more about Design Thinking (DT)
Take a deep dive into Design Thinking (DT) with our course Design Thinking: The Ultimate Guide .
Some of the world’s leading brands, such as Apple, Google, Samsung, and General Electric, have rapidly adopted the design thinking approach, and design thinking is being taught at leading universities around the world, including Stanford d.school, Harvard, and MIT. What is design thinking, and why is it so popular and effective?
Design Thinking is not exclusive to designers —all great innovators in literature, art, music, science, engineering and business have practiced it. So, why call it Design Thinking? Well, that’s because design work processes help us systematically extract, teach, learn and apply human-centered techniques to solve problems in a creative and innovative way—in our designs, businesses, countries and lives. And that’s what makes it so special.
The overall goal of this design thinking course is to help you design better products, services, processes, strategies, spaces, architecture, and experiences. Design thinking helps you and your team develop practical and innovative solutions for your problems. It is a human-focused , prototype-driven , innovative design process . Through this course, you will develop a solid understanding of the fundamental phases and methods in design thinking, and you will learn how to implement your newfound knowledge in your professional work life. We will give you lots of examples; we will go into case studies, videos, and other useful material, all of which will help you dive further into design thinking. In fact, this course also includes exclusive video content that we've produced in partnership with design leaders like Alan Dix, William Hudson and Frank Spillers!
This course contains a series of practical exercises that build on one another to create a complete design thinking project. The exercises are optional, but you’ll get invaluable hands-on experience with the methods you encounter in this course if you complete them, because they will teach you to take your first steps as a design thinking practitioner. What’s equally important is you can use your work as a case study for your portfolio to showcase your abilities to future employers! A portfolio is essential if you want to step into or move ahead in a career in the world of human-centered design.
Design thinking methods and strategies belong at every level of the design process . However, design thinking is not an exclusive property of designers—all great innovators in literature, art, music, science, engineering, and business have practiced it. What’s special about design thinking is that designers and designers’ work processes can help us systematically extract, teach, learn, and apply these human-centered techniques in solving problems in a creative and innovative way—in our designs, in our businesses, in our countries, and in our lives.
That means that design thinking is not only for designers but also for creative employees , freelancers , and business leaders . It’s for anyone who seeks to infuse an approach to innovation that is powerful, effective and broadly accessible, one that can be integrated into every level of an organization, product, or service so as to drive new alternatives for businesses and society.
You earn a verifiable and industry-trusted Course Certificate once you complete the course. You can highlight them on your resume, CV, LinkedIn profile or your website .
All open-source articles on Design Thinking (DT)
What is design thinking and why is it so popular.
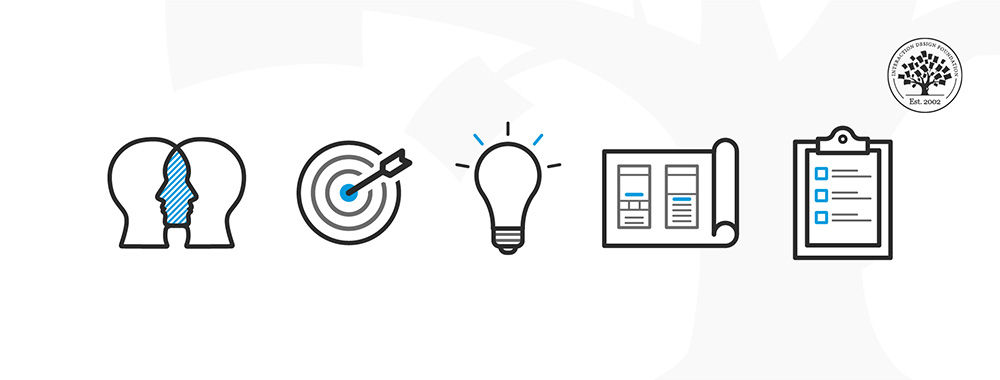
- 1.6k shares
Personas – A Simple Introduction
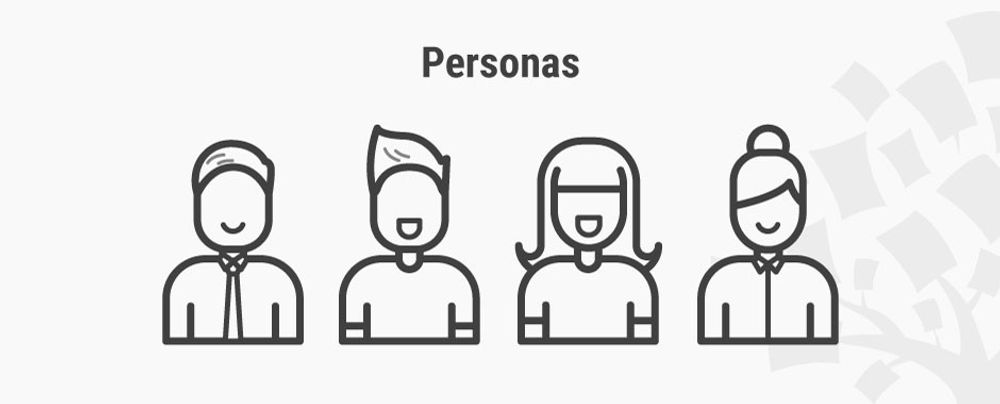
- 1.5k shares
Stage 2 in the Design Thinking Process: Define the Problem and Interpret the Results
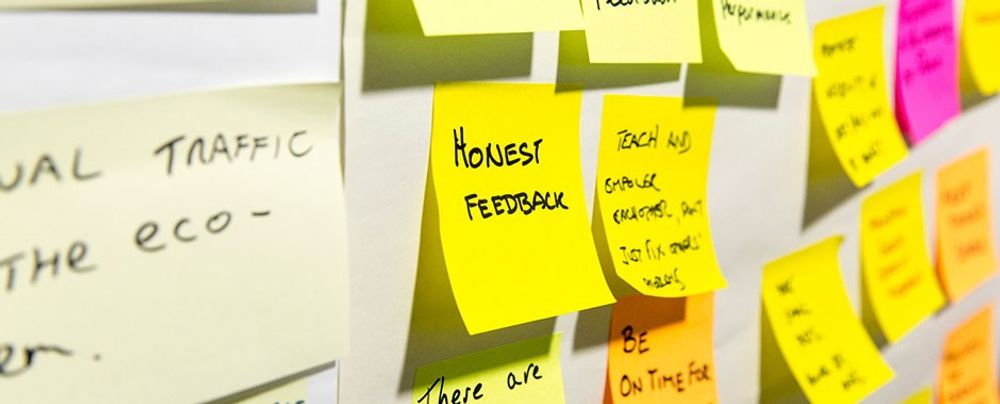
- 1.3k shares
What is Ideation – and How to Prepare for Ideation Sessions
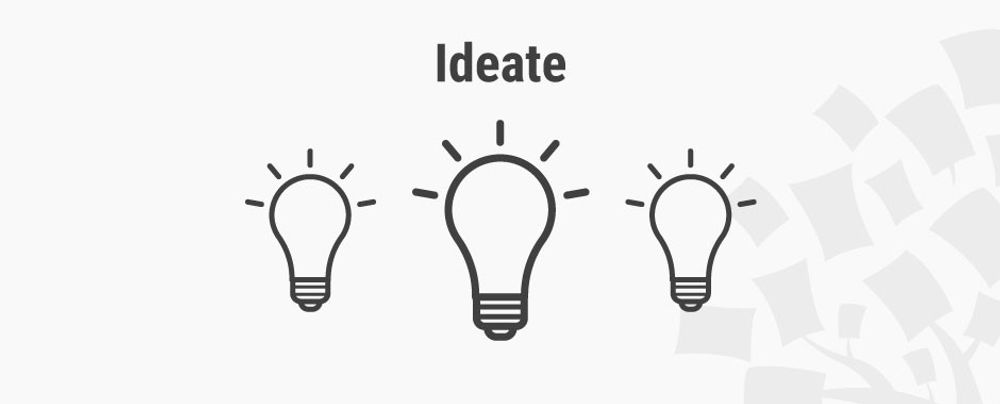
Affinity Diagrams: How to Cluster Your Ideas and Reveal Insights
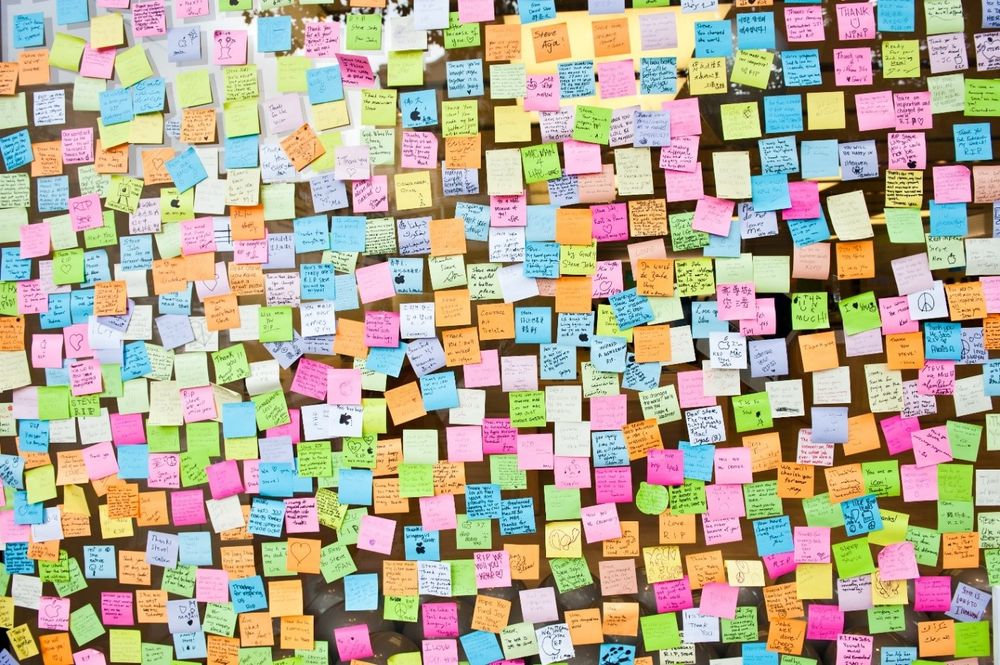
- 1.2k shares
- 2 years ago
Stage 4 in the Design Thinking Process: Prototype
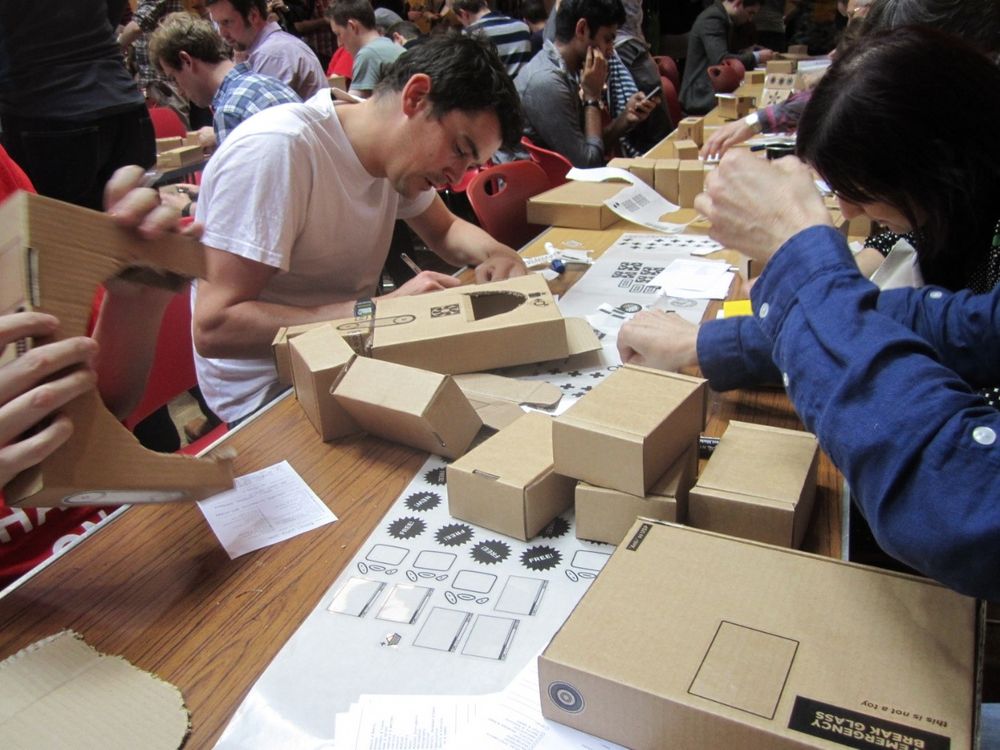
- 3 years ago
Stage 3 in the Design Thinking Process: Ideate
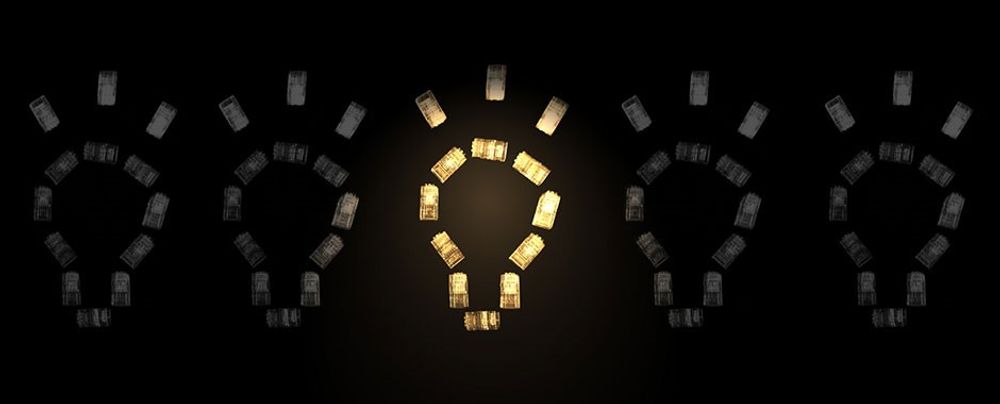
- 4 years ago
Stage 1 in the Design Thinking Process: Empathise with Your Users
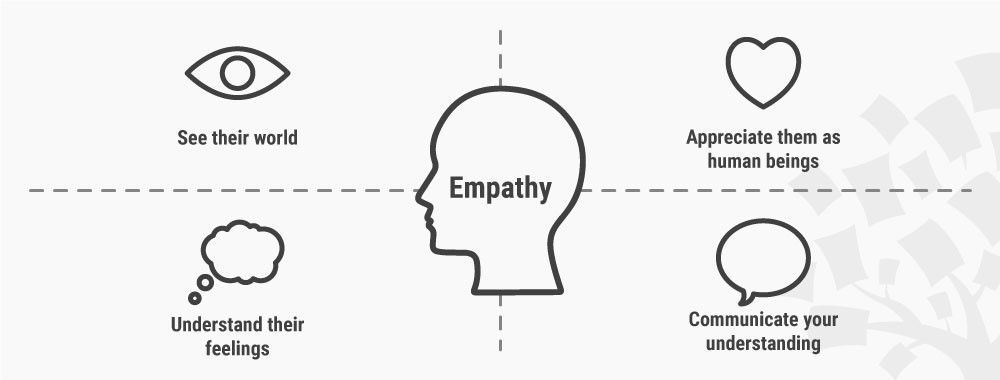
Empathy Map – Why and How to Use It
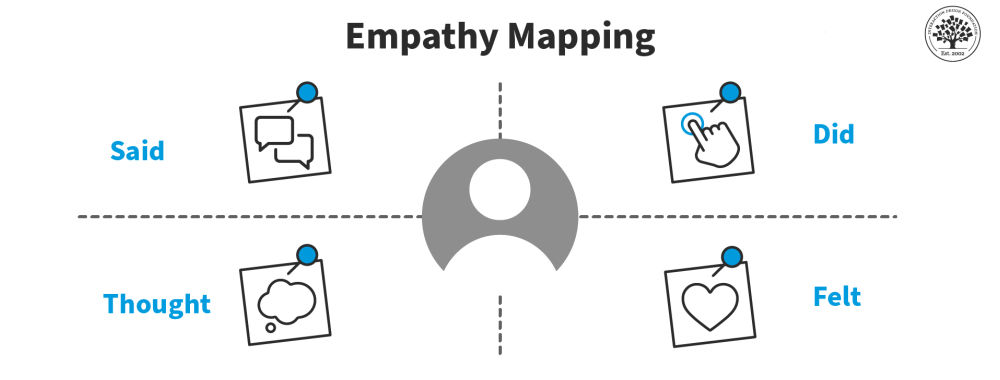
What Is Empathy and Why Is It So Important in Design Thinking?
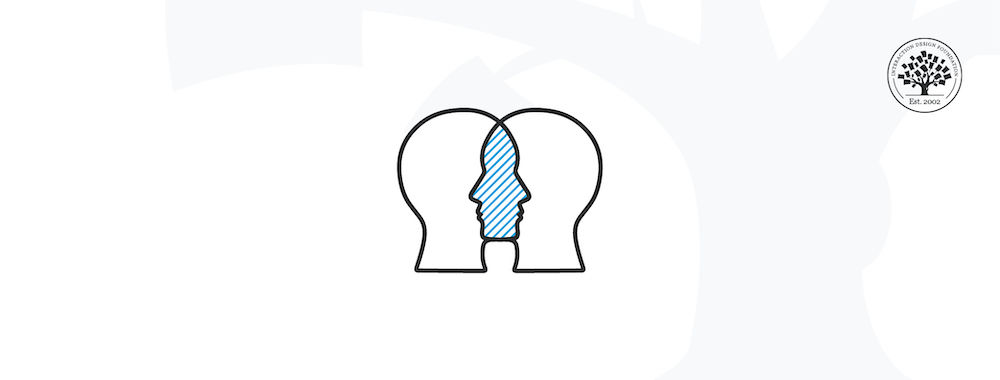
10 Insightful Design Thinking Frameworks: A Quick Overview
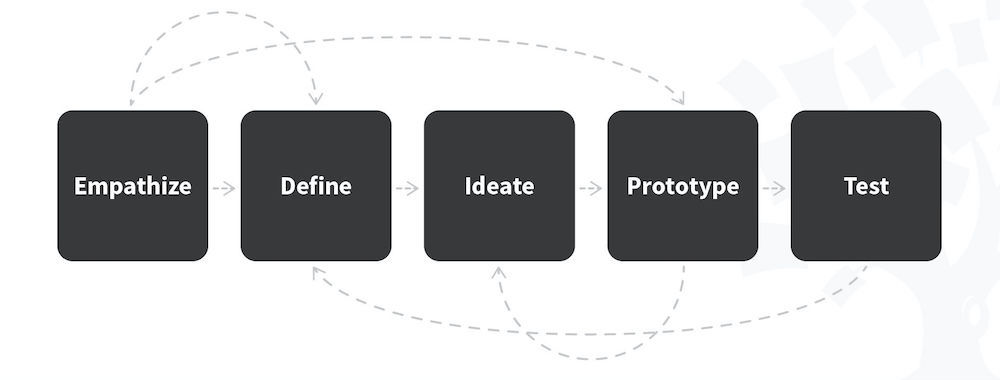
Define and Frame Your Design Challenge by Creating Your Point Of View and Ask “How Might We”
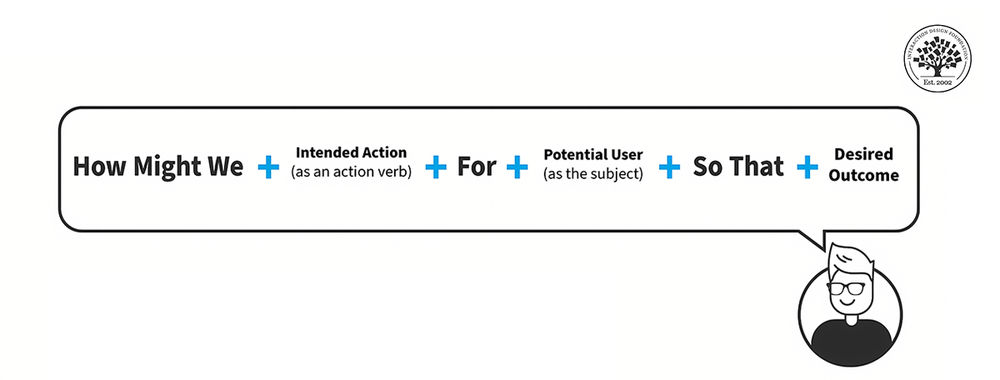
Design Thinking: Get Started with Prototyping
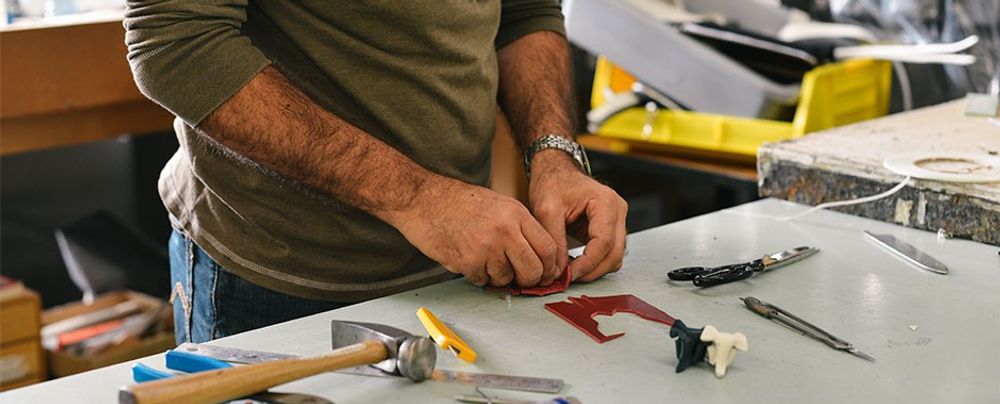
- 1.1k shares
5 Common Low-Fidelity Prototypes and Their Best Practices
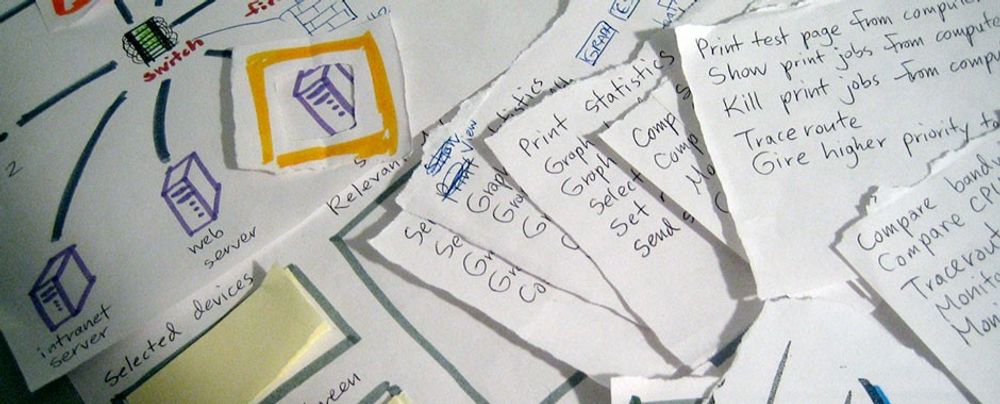
Design Thinking: New Innovative Thinking for New Problems
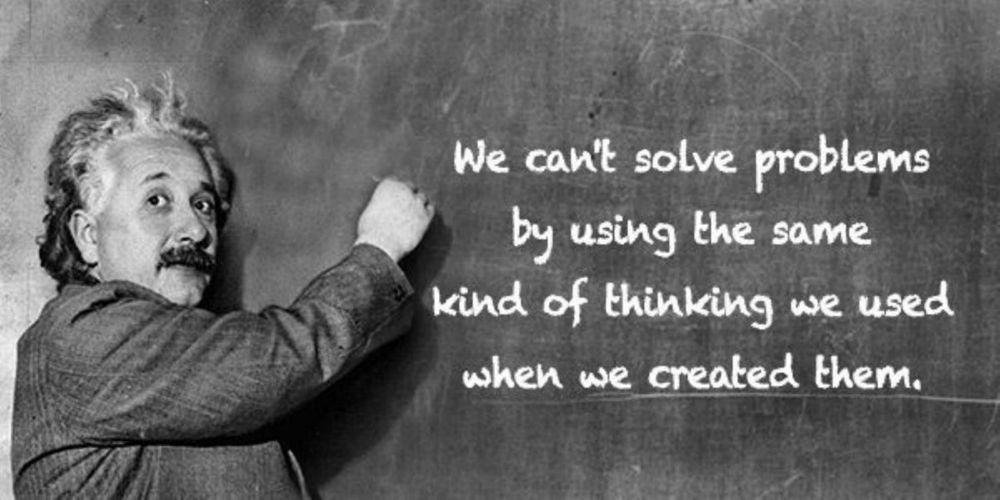
The History of Design Thinking
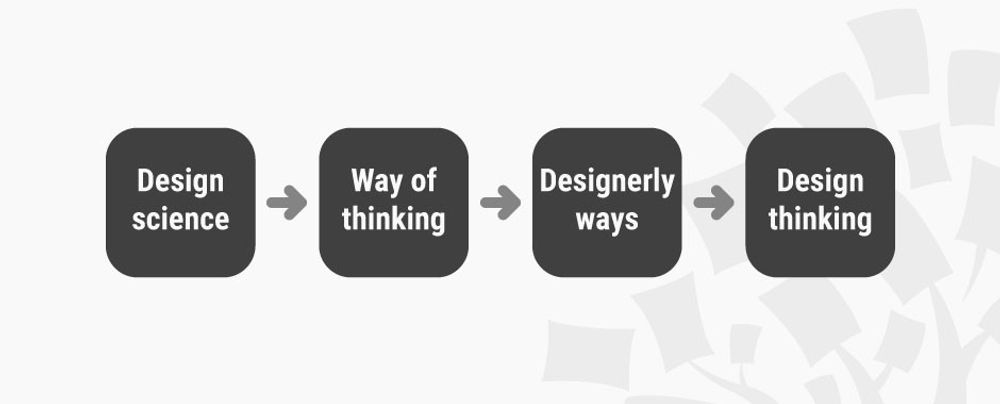
Test Your Prototypes: How to Gather Feedback and Maximize Learning
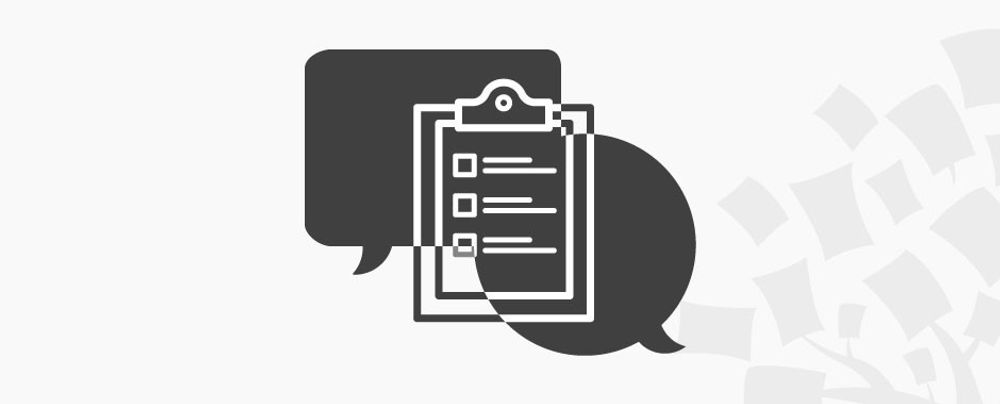
The Ultimate Guide to Understanding UX Roles and Which One You Should Go For
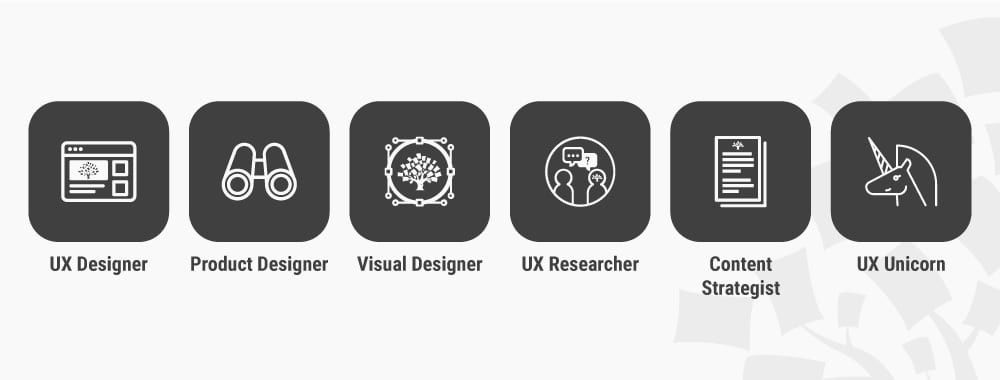
- 10 mths ago
Stage 5 in the Design Thinking Process: Test
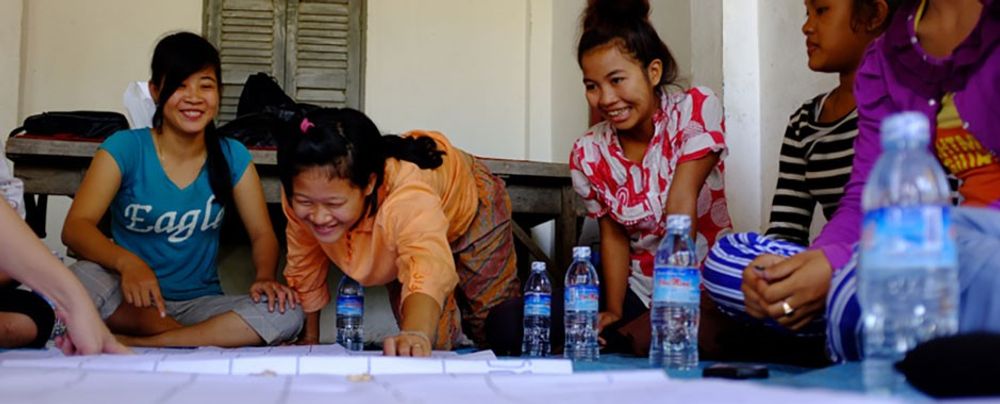
What Are Wicked Problems and How Might We Solve Them?
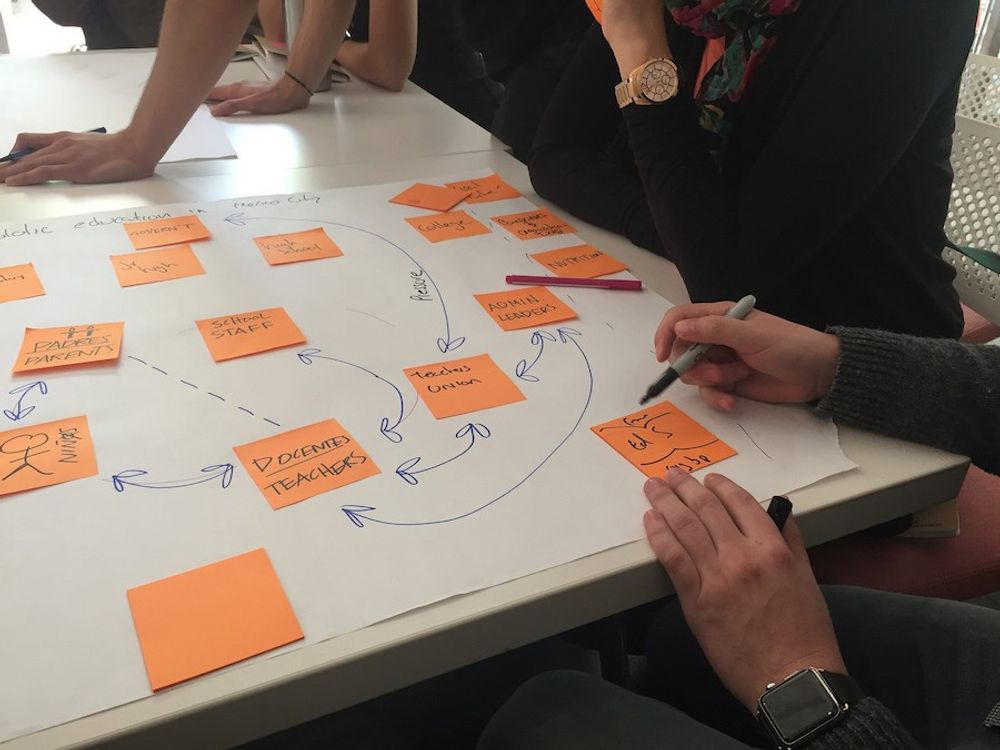
Open Access—Link to us!
We believe in Open Access and the democratization of knowledge . Unfortunately, world-class educational materials such as this page are normally hidden behind paywalls or in expensive textbooks.
If you want this to change , cite this page , link to us, or join us to help us democratize design knowledge !
Privacy Settings
Our digital services use necessary tracking technologies, including third-party cookies, for security, functionality, and to uphold user rights. Optional cookies offer enhanced features, and analytics.
Experience the full potential of our site that remembers your preferences and supports secure sign-in.
Governs the storage of data necessary for maintaining website security, user authentication, and fraud prevention mechanisms.
Enhanced Functionality
Saves your settings and preferences, like your location, for a more personalized experience.
Referral Program
We use cookies to enable our referral program, giving you and your friends discounts.
Error Reporting
We share user ID with Bugsnag and NewRelic to help us track errors and fix issues.
Optimize your experience by allowing us to monitor site usage. You’ll enjoy a smoother, more personalized journey without compromising your privacy.
Analytics Storage
Collects anonymous data on how you navigate and interact, helping us make informed improvements.
Differentiates real visitors from automated bots, ensuring accurate usage data and improving your website experience.
Lets us tailor your digital ads to match your interests, making them more relevant and useful to you.
Advertising Storage
Stores information for better-targeted advertising, enhancing your online ad experience.
Personalization Storage
Permits storing data to personalize content and ads across Google services based on user behavior, enhancing overall user experience.
Advertising Personalization
Allows for content and ad personalization across Google services based on user behavior. This consent enhances user experiences.
Enables personalizing ads based on user data and interactions, allowing for more relevant advertising experiences across Google services.
Receive more relevant advertisements by sharing your interests and behavior with our trusted advertising partners.
Enables better ad targeting and measurement on Meta platforms, making ads you see more relevant.
Allows for improved ad effectiveness and measurement through Meta’s Conversions API, ensuring privacy-compliant data sharing.
LinkedIn Insights
Tracks conversions, retargeting, and web analytics for LinkedIn ad campaigns, enhancing ad relevance and performance.
LinkedIn CAPI
Enhances LinkedIn advertising through server-side event tracking, offering more accurate measurement and personalization.
Google Ads Tag
Tracks ad performance and user engagement, helping deliver ads that are most useful to you.
Share Knowledge, Get Respect!
or copy link
Cite according to academic standards
Simply copy and paste the text below into your bibliographic reference list, onto your blog, or anywhere else. You can also just hyperlink to this page.
New to UX Design? We’re Giving You a Free ebook!
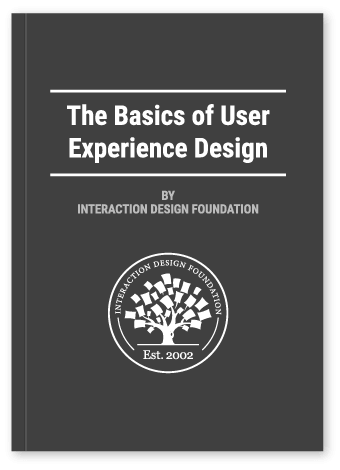
Download our free ebook The Basics of User Experience Design to learn about core concepts of UX design.
In 9 chapters, we’ll cover: conducting user interviews, design thinking, interaction design, mobile UX design, usability, UX research, and many more!
Research on Teaching Design of "System of Systems Design and Evaluation" Course Based on System Thinking
New citation alert added.
This alert has been successfully added and will be sent to:
You will be notified whenever a record that you have chosen has been cited.
To manage your alert preferences, click on the button below.
New Citation Alert!
Please log in to your account
Information & Contributors
Bibliometrics & citations, index terms.
Information systems
Information systems applications
Recommendations
Research on college classroom teaching evaluation system and evaluation method.
The college classroom teaching is an important part of the quality of undergraduate education teaching, it plays a key role in improving the quality of undergraduate education comprehensively, therefore, it has the important significance to improve ...
Teaching how to teach computational thinking
Computational Thinking is argued to be an essential skill for the workforce of the 21st century. As a skill, Computational Thinking should be taught in all schools, employing computational ideas integrated into other disciplines. Up until now, questions ...
Teaching and assessing an interdisciplinary science of design pilot course
The role of design and creativity is well established in many disciplines in science, engineering and art. However, in computing science disciplines, specifically in the development of software systems and information technology, the computing ...
Information
Published in.

Association for Computing Machinery
New York, NY, United States
Publication History
Permissions, check for updates.
- Research-article
- Refereed limited
Contributors
Other metrics, bibliometrics, article metrics.
- 0 Total Citations
- 0 Total Downloads
- Downloads (Last 12 months) 0
- Downloads (Last 6 weeks) 0
View Options
Login options.
Check if you have access through your login credentials or your institution to get full access on this article.
Full Access
View options.
View or Download as a PDF file.
View online with eReader .
HTML Format
View this article in HTML Format.
Share this Publication link
Copying failed.
Share on social media
Affiliations, export citations.
- Please download or close your previous search result export first before starting a new bulk export. Preview is not available. By clicking download, a status dialog will open to start the export process. The process may take a few minutes but once it finishes a file will be downloadable from your browser. You may continue to browse the DL while the export process is in progress. Download
- Download citation
- Copy citation
We are preparing your search results for download ...
We will inform you here when the file is ready.
Your file of search results citations is now ready.
Your search export query has expired. Please try again.
Faculty of Agriculture
- Agricultural Economics
- Food Science, Nutrition & Technology
- Land Resource Management & Agricultural Technology
- Plant Science & Crop Protection
Faculty of Built Environment
- Art and Design
- Architecture
- Real Estate, Construction Management & Quantity Surveying
- Urban and Regional Planning
FACULTY OF BUSINESS & MANAGEMENT SCIENCES
- Business Administration
- Finance and Accounting
- Management Science and Project Planning
FACULTY OF EDUCATION
- Educational Management, Policy and Curriculum Studies
- Educational Communication and Pedagogical Studies
- Physical Education and Sport
- Educational Foundations
- Educational and Distance Studies
- FACULTY OF LAW
FACULTY OF ENGINEERING
- Mechanical and Manufacturing Engineering
- Civil and Construction Engineering
- Electrical and Information Engineering
- Environmental and Biosystems Engineering
- Geospatial and Space Technology.
FACULTY OF ARTS AND SOCIAL SCIENCES
- Anthropology, Gender and African Studies
- Diplomacy and International Studies
- Economics and Development Studies
- Geography, Population and Environmental Studies
- History and Archaeology
- Journalism and Mass Communication
- Library and Information Science
- Linguistics and Languages
- Philosophy and Religious Studies
- Political Science and Public Administration
- Sociology, Social Work and African Women Studies
FACULTY OF HEALTH SCIENCES
- Dental sciences
- Nursing Sciences
- Public and Global Health
- Human Anatomy & Physiology
- Clinical Medicine & Therapeutics
- Paediatrics and Child Health
- Obstetrics & Gynecology
- Human Pathology
- Diagnostic Imaging & Radiation Medicine
- Medical Microbiology and Immunology
- Ophthalmology
FACULTY OF SCIENCE & TECHNOLOGY
- Computing and Informatics
- Mathematics
- Earth & Climate Science
- Biochemistry
FACULTY OF VETERINARY MEDICINE
- Public Health,Pharmacology & Toxicology
- Veterinary Anatomy & Physiology
- Animal Production
- Clinical Studies
- Veterinary Pathology, Microbiology & Parasitology
RESEARCH INSTITUTES
- Wangari Maathai Institute for Peace and Environmental Studies
- Institute of Nuclear Science & Technology
- The Institute for Climate Change and Adaptation
- Kenya Aids Vaccine Institute
- Institute of Diplomacy and International Studies
- Institute of Anthropology, Gender and African Studies
- Institute of Tropical & Infectious Diseases
- East African Kidney Institute
- Population Studies and Research Institute
- Institute for Development Studies
- Confucius institute
RESEARCH CENTRES
- African Women's Studies Centre
- Center for Epidemiological Modelling and Analysis (CEMA)
- Centre For Biotechnology & Bioinformatics
- Centre for Advanced Studies in Environmental Law & Policy
- Centre for Translation and Interpretation
- Virtual Tour
- Data Privacy
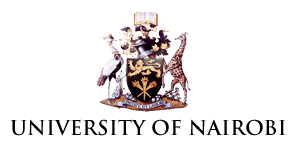
C4DLab Conducts Cross-Faculty Innovation Training on Design Thinking and Problem-Based Learning
The C4DLab is running a week-long training program for faculty on Design Thinking and Problem-Based Learning, innovative problem-solving approaches. The training, aptly dubbed “Innovation Training” for revolutionary approach to solving real-world problems, is currently underway in the PhD room of the Department of Computing and Informatics.
The training has attracted participants from various faculties within the University of Nairobi and is set to conclude on Friday, September 6, 2024.
This training programme aligns with Afretech's Innovation and Entrepreneurship Pillar, headed by Dr. Sam Ruhiu, the primary facilitator of the workshop.
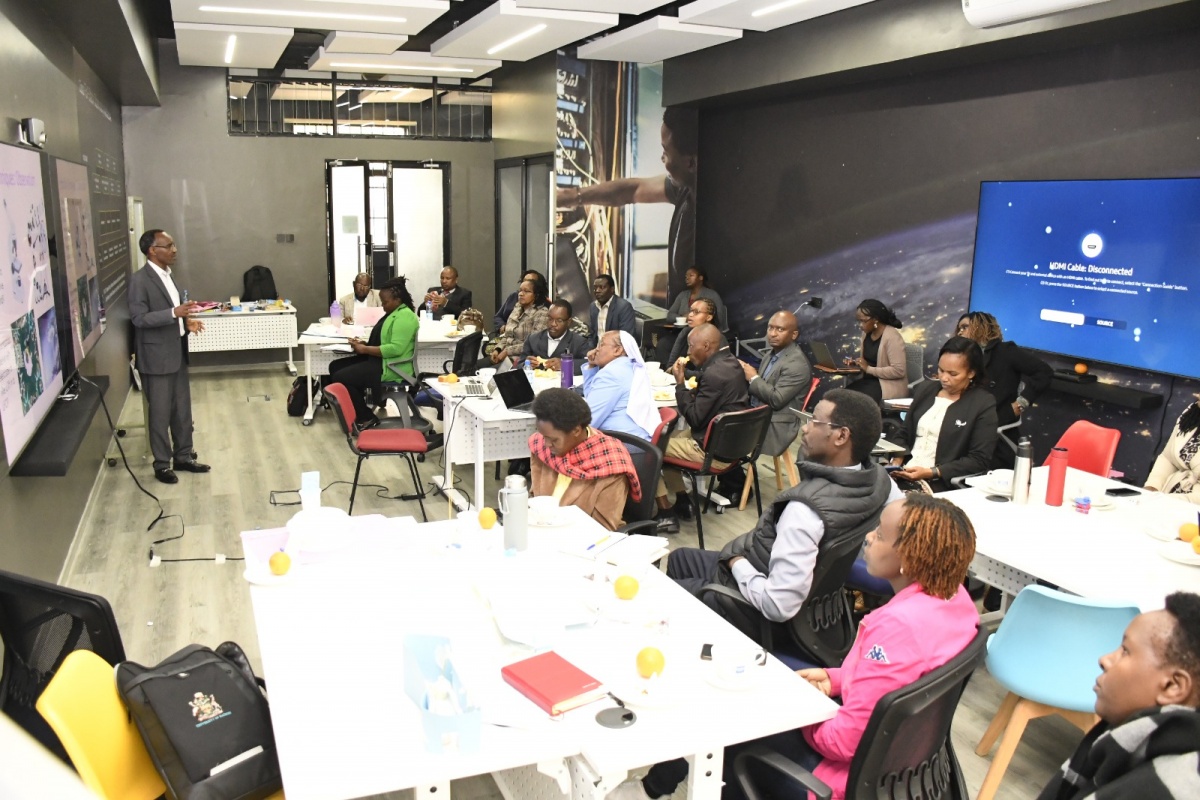
Dr. Ruhiu emphasized the need for a paradigm shift among university faculty, stressing the need to move beyond mere scholarly research results and translate them into practical innovations that address real-world needs. He highlighted the importance of a mental shift towards entrepreneurship, which involves creating products and services that meet consumer demands as a way of completing the innovation pipeline.
Historically, many valuable research projects by both faculty and students have stalled at the research results stage, failing to progress to innovation and entrepreneurship. This training aims to rectify this trend.
The morning session provided a foundation for Design Thinking, covering its historical development and case studies from renowned global academic institutions like Stanford, MIT and University of Colorado in the U.S., and Aalto in Finland.
The session also explored notable innovation failures, such as those by Thomas Edison (the inventor of the light bulb), AT&T, and Warner Annex. These failures were often attributed to a techno-centric focus rather than a user-needs-driven approach.
The training's goal is to equip educators with practical tools and strategies to foster creativity and critical thinking in the classroom. By enhancing faculty's ability to drive innovation, the training seeks to prepare students for future problem-solving challenges.
The specific objectives of the training include:
- Learning how to apply the Design Thinking process to identify and solve real-world problems.
- Encouraging students to develop innovative solutions through Design Thinking.
- Empowering educators to use Problem-Based Learning to create student-centered learning environments that foster active engagement, collaboration, and critical thinking.
The second session, co-facilitated by Dr. Ruhiu, Mr. Peter Oketch and Ms. Caroline Jelagat, involved a number of team-building exercises where participants from different faculties worked together to construct a tower. The exercises demonstrated the importance of teamwork, a crucial aspect of Design Thinking.
The session concluded with a reflection time, during which participants considered the most challenging aspects of the task, individual roles, and the emergence of leadership within the group. Then there was Experience Design, where a two-member team designed a gift-giving experience for the partner in turn and each evaluated the experience through discovery questions.
We are able to share the photos, or any other material, should your office need them. much as I think your cameraman took more professional photos. (We only had a phone.)
Instructively, since the event is on for the next few days, we are going to build on the above story and will certainly share the latest version at an appropriate time.
As we appreciate the role your office plays in amplifying the university's voice on important matters, we would be more than glad if your office could run this story to give it the necessary publicity.
We are looking forward to collaborating with you even more for future events.
Read related articles here


- Back Issues
- Letters Policy
Projects selected for dB-SERC Course Transformation Awards
The Discipline-Based Science Education Research Center (dB-SERC) has awarded 12 Course Transformation Awards to faculty in natural sciences.
Since 2014, dB-SERC has supported natural sciences faculty members in developing projects to transform the way classes are taught by adopting evidence-based teaching practice to improve student learning outcomes.
Award recipients receive funds for equipment, student support or summer salary for faculty. Two mentor-mentee awards also were given out to support classroom innovation projects conducted by students and faculty working together.
Course Transformation Awards
Young Ahn, Department of Biological Sciences: Designing a high-structure course combining frequent low-stakes assessments with inclusive teaching for a large-enrollment introductory biology class
This proposal aims to test the “heads and hearts” hypothesis which suggests that both students’ cognitive (heads) and affective (hearts) learning experiences must be purposefully constructed in classroom environments. This project will investigate whether a course structure that combines frequent low-stakes assessments (heads) and inclusive teaching (hearts) can improve student performance and reduce achievement gaps in a large-enrollment introductory biology course thereby promoting retention in STEM.
Anusha Balangoda, Department of Geology and Environmental Science : Use of a Collaborative Online Reading Platform for Pre-class Reading Assignments in a Large Enrollment First-Year Undergraduate Class
The proposed work seeks funding to implement pre-class reading assignments through a social annotation platform allowing active reading on assigned course materials outside the class. A free social platform, Perusall, provides an interactive experience for students to engage with peers asynchronously and facilitates a space to teach and learn from peers. This collaborative social platform allows students to work on assignments outside the classroom to promote productive discussions and produce high-quality peer interactions.
Seth Childers, Department of Chemistry: Development of Interdisciplinary Courses for a New Chemical Biology Major
In the Department of Chemistry, the PI is proposing a chemical biology major, including two new lecture courses and one laboratory course, proposed to launch in Fall 2025 or 2026. This timeline allows them to craft a curriculum while deploying evidence-based learning practices to enhance job readiness. Based on student surveys, the program aims to accommodate approximately 48 majors annually and engage non-majors as a desirable scientific elective campus wide.
Russell Clark and Aidan Payton, Department of Physics & Astronomy: Gender Equity in Introductory Physics Lab Group Roles
This is a continuation of a dB-SERC award from 2020 (Development of Teacher Guides and Rubrics for Introductory Physics Labs). The original plan for that award was to develop better rubrics and other materials to help the TA graders provide more valuable feedback to the students. However, the University was forced into quarantine midway through the first semester of the project, and so the character of it changed. They know from a previous study that student groups tend to have gender bias in which men tend to work with the experimental apparatus and women are relegated to secretarial roles (recording data, writing the report, etc.). They attempted to mitigate this by asking the students to cycle through the roles week to week so that each student would get to participate in each role multiple times.
Erika Fanselow, Department of Neuroscience: Incorporating digital and physical 3D brain models into interactive online and in-class activities to enhance student engagement and mastery in neuroanatomy courses
The goal of this course transformation is to develop interactive, online and in-class exercises that incorporate digital and printed 3D models of nervous system structures. These 3D model-based exercises and in-class activities are intended to enhance students’ visualization and conceptualization of neuroanatomical structures. The rationale for this course transformation proposal is based on the fact that neuroanatomy students are commonly overwhelmed by the complexity of the nervous system, resulting in a condition Jozefowicz (1994) referred to as “neurophobia,” which he concluded actually keeps students from choosing fields such as neurology.
Sean Garrett-Roe, Department of Chemistry: Activity redesign and mindset intervention based on growth-oriented testing in Chem-0110 General Chemistry I
“Grading for Growth” is a movement to encourage students to embrace deeper intellectual engagement with their studies by revolutionizing the way that their learning is assessed. Student-focused active learning pedagogies, such as Process Oriented Guided Inquiry Learning (POGIL), are well-established; student-focused assessments, on the other hand, are a new frontier. The PIs have formulated, implemented and assessed a student-focused assessment system that they call “Growth-Oriented Testing.” As successful as the system has been, the assessment results have illuminated ways in which their in-class materials have not optimally supported students, and the student opinion surveys suggest ways in which they have not optimally framed the learning process. As a result, students may not get the full benefits of the learning environment. A long-range goal of their teaching is to help students embrace a life of growth and learning; they want the students to learn both Chemistry and the metacognitive and metaemotional skills they need to succeed beyond the Chemistry classroom.
Sean Gess, Department of Biological Sciences: Supporting richer class-wide discussion and promoting the use of scientific argumentation in Foundations of Biology laboratory courses
This project focuses on class-wide discussion in a guided, authentic research lab. In this course students engage in science education by performing authentic research science to address active research questions being investigated within the department. The course is designed to mimic the research process, including discussions of data to try and understand it better. These discussion-based activities often struggle to support the learning objectives due to low participation from students or students not really listening and engaging with others during the discussions. To improve these discussions, they have previously introduced an explicit framing to attempt to help students understand the norms around this activity, normalize it as a professional practice, and encourage engagement and participation. This approach to science learning has shown gains in critical thinking skills and supports epistemic learning of STEM content.
Burhan Gharaibeh, Natasha Baker and Bridget Deasy, Department of Biological Sciences: Enhancing student engagement in anatomy and physiology courses through regenerative medicine primary science literature
Students of anatomy and physiology in different majors often report difficulty in these courses due to the need for memorizing lists of structures and comprehending complex physiological processes. They have preliminary data demonstrating that adding discussions of current, clinically relevant therapies and biotechnology articles related to regenerative medicine studies were effective in enhancing the biology student’s engagement during anatomy lectures. More importantly, the addition of these discussions to the curriculum appeared to improve exam grades.
Melanie Good and Eric Swanson, Department of Physics & Astronomy: The Use of Comprehensive PACE (Pseudoscience and Conspiracy-theory Education) in Physics and Society
Phys0087: Physics and Society was a course developed by Eric Swanson to help students examine the conceptual foundations of modern science with the goal of understanding how science affects our daily lives and our impact on the environment. At the intersection of science and society lies the issue of popular belief in the claims of pseudoscience and conspiracy theories. These beliefs are fairly common and often can be difficult to dislodge with education in science alone. However, past work has shown that explicit instruction on topics related to pseudoscience and conspiracy theory beliefs may be effective in reducing endorsement of these beliefs. The PIs have seen this among their own students, based on pilot data and data from a previous dB-SERC Course Transformation Award. The success of their earlier work has captured the attention not only of our university media, but also the Lilienfeld Alliance, a group of higher education professionals across the nation that is committed to promoting critical thinking skills in the face of the claims of pseudoscience, who invited them to join their cause. With the momentum they have built, they are inspired to more comprehensively overhaul Phys0087: Physics and Society to expand upon their original transformation. Their new proposed course transformation would extend the pseudoscience module into a comprehensive PACE (Pseudoscience and Conspiracy-theory Education) curriculum in Phys0087–Physics and Society during the 2024-2025 school year.
Edison Hauptman and Jeffrey Wheeler, Department of Mathematics: Contract Grading in Calculus 2
In summer 2024, Edison Hauptman’s section of Analytic Geometry & Calculus 2 (Math 0230) was taught with a different set of assignments and grading structure. The grading structure for the class resembled a contract between the instructor and their students: the instructor provided many different assignments, and for a student to earn a desired grade, they had to score enough points on various assignments of their choice to reach that grade’s point threshold. This course structure can have many variations and is called a “grading contract.” Compared to the current (default) course structure for Calculus courses at the University of Pittsburgh, a grading contract is a more equitable way to evaluate a diverse set of students, allows the instructor to be more accommodating to students without sacrificing the course’s rigor, and encourages more student buy-in. This project develops and evaluates a set of assignments offered to students in Hauptman’s Summer 2024 12-week section of Math 0230 and focuses on mathematical skills emphasized in each assignment.
Zuzana Swigonova, Department of Biological Sciences: Combining computer visualizations with 3D printed models to engage students in active study of molecular structure and function
All biological processes in a living system depend on proper functioning of molecules. Understanding the principles of molecular structure, the three-dimensional spatial arrangements of atoms and functional groups that allow for intra- and intermolecular interactions, is crucial for grasping the fundamentals of structure-function relationships. Despite the many benefits of physical 3D models, printing intricate biological molecules has several limitations, such as low level of atomic detail in complex structures, depiction of a single static molecular representation, and labor-intensive post-printing processing. Computer visualization allows for the development of abundant resources that complement physical models with no added material cost. They propose to develop teaching resources using computer visualization to supplement the physical 3D models.
Margaret Vines, Department of Chemistry: Learning to learn chemistry
The purpose of this project is to help students learn. Most students come to college with the desire to learn. They want to be successful and learn the material presented to them in their classes. Unfortunately, many of them engage in activities that do not help with their learning. The PI’s goal is to help students begin to learn how to learn. They will do this as part of their regular lecture and recitation in general Chemistry. They will educate them about learning techniques and explain why they will aid in their learning. They will then demonstrate these techniques in class, and the students will be given opportunities to use these techniques inside and outside the lecture and recitation. Finally, they will encourage their students to develop those techniques for use in their other classes.
Mentor/Mentee Award
Mentor: Anusha Balangoda / Mentee: Beth Ann Eberle. Department of Geology and Environmental Science: Use of Cooperative Learning Approach in Recitations to Untangle Pressing Environmental Issues in Introductory Environmental Science Class
Cooperative learning is a student-centered active learning strategy in which a small group of students is responsible for their own success and that of their team by holding themselves accountable for the process and outcomes of the activities. In this project, they propose to use a cooperative learning strategy in the GEOL 0840 Introductory Environmental Science course, which is a large enrollment three-credit class, and both lectures and recitations are required.
Mentor: Ben Rottman / Mentee: Rebecca McGregor. Department of Psychology; Learning Research and Development Center: Using a Consulting Model and Project-Based Learning to Teach Psychology Research Methods
In the field of psychology, research methods form the foundation of students’ knowledge during the remainder of their undergraduate degree and beyond. Students in PSY 0036: Research Methods Lecture at the University of Pittsburgh have three course objectives: learn how to read, interpret and discuss research design and conclusions, learn how to critique research, and learn how to design valid research. There are currently few opportunities for students to apply this knowledge to real-world experiences, as this is an introductory course in which students have not yet developed the skills to analyze and interpret their own data. Thus, this course design through the dB-SERC would provide a semester-long collaborative assignment in which students would develop a project proposal to investigate a real-world research problem for a fictional client.

IMAGES
VIDEO
COMMENTS
The article discusses design thinking as a process and mindset for collaboratively finding solutions for wicked problems in a variety of educational settings. Through a systematic literature ...
The article discusses design thinking as a process and mindset for collaboratively finding solutions for wicked problems in a variety of educational settings. Through a systematic literature review the article organizes case studies, reports, theoretical reflections, and other scholarly work to enhance our understanding of the purposes, contexts, benefits, limitations, affordances, constraints ...
Design Thinking in Education. Design Thinking is a mindset and approach to learning, collaboration, and problem solving. In practice, the design process is a structured framework for identifying challenges, gathering information, generating potential solutions, refining ideas, and testing solutions. Design Thinking can be flexibly implemented ...
Daniela Gachago is an associate professor at the Centre for Innovative Educational Technology, at the Cape Peninsula University of Technology. Her research focuses on academic staff development on the use of emerging technologies to transform teaching and learning in higher education, with a particular focus on socially just pedagogies such as digital storytelling.
the adoption of design thinking in education. For example, Goldman, Kabayadondo, Royalty, Carroll, and Roth (2014) stated that in in over 60 US universities and colleges, design thinking is taught through workshops, supplemental training, courses, or degree programs. Similarly, Callahan (2019) observed that design thinking
Research in Education & Design. REDlab's mission is to conduct research to inform our understanding of design thinking in K-12, undergraduate and graduate educational settings. Design thinking focuses on needfinding, challenging assumptions, generating a range of possibilities, and learning through targeted stages of iterative prototyping.
research, we used a mixed-method sequential explanatory research design (Creswell and Clark 2018; Ivankova, Creswell, and Stick 2006). The research was determined to be exempt from further review ... DESIGN THINKING IN HIGHER EDUCATION: OPPORTUNITIES AND CHALLENGES FOR DECOLONIZED LEARNING Lake, Danielle, Wen Guo, Elizabeth Chen, and Jacqui ...
Design and design thinking are vital to creativity and innovation, and have become increasingly important in the current movement of developing and implementing integrated STEM education. In this editorial, we build on existing research on design and design thinking, and discuss how students' learning and design thinking can be developed through design activities in not only engineering and ...
This study aims to provide an overview of design thinking education research regarding research outcomes, most popular publication sites, most involved researchers, and countries. Based on the findings obtained. The main results suggest that there has been an increasing trend in the amount of research conducted on the subject of design thinking ...
European Journal of Education covers all areas of educational research from global contributors, ... The findings highlight the benefits of the design thinking approach to skills development and point to the impact of the course content and layout and certain design thinking tools applied in developing 21st century skills.
Education needs new ways to prepare individuals and societies for the multitude of changing challenges in the twenty-first century. In today's world—characterized by digitization, increasing speed, and complexity—design thinking has established itself as a powerful approach to human-centered innovation that can help address complicated problems and guide change in all areas of life.
Design thinking is regarded as an essential way to cultivate 21st century competency and there has been a concomitant rise of needs and interest in introducing K-12 students to design thinking. This study aimed to review high-qualified empirical studies on design thinking integrated learning (DTIL) in K-12 education and explore its future research perspectives.
Design thinking research. New York: Springer; 2014. pp. 79-96. 24. Liedtka J, Bahr KJ. Assessing design thinking's impact: Report on the development of a new instrument. Darden Working Paper Series; 2019. 25. Guo W, Lake D, Cox M, Chen E, McLaughlin JE. De-disciplining and democratizing design thinking pedagogies in higher education.
Abstract and Figures. Design Thinking is an innovative, creative and human-centered process and mindset that employs collaborative multidisciplinary teams in order to generate user- focused ...
Chapter. The nine chapters in this book provided rich perspectives on ways of thinking about and enacting educational design. Throughout the book, the authors shared examples of prominent design processes in education, along with how teachers, system leaders, university instructors, and students are engaging in design in varied contexts.
This book therefore explores, through eight chapters, how desi gn thinking. vocabulary can be interpreted and employed in educational cont exts. Chap. 1. examines the role that design thinking can ...
(DOI: 10.1515/EDU-2019-0022) Abstract The article discusses design thinking as a process and mindset for collaboratively finding solutions for wicked problems in a variety of educational settings. Through a systematic literature review the article organizes case studies, reports, theoretical reflections, and other scholarly work to enhance our understanding of the purposes, contexts, benefits ...
%PDF-1.4 1 0 obj /Title (þÿ) /Creator (þÿwkhtmltopdf 0.12.2.1) /Producer (þÿQt 4.8.6) /CreationDate (D:20151102031214Z) >> endobj 3 0 obj /Type /ExtGState /SA true /SM 0.02 /ca 1.0 /CA 1.0 /AIS false /SMask /None>> endobj 4 0 obj [/Pattern /DeviceRGB] endobj 6 0 obj /Type /XObject /Subtype /Image /Width 2499 /Height 5101 /BitsPerComponent 8 /ColorSpace /DeviceRGB /Length 7 0 R /Filter ...
Design thinking is both a method and a mindset. What makes design thinking unique in comparison to other frameworks such as project based learning, is that in addition to skills there is an emphasis on developing mindsets such as empathy, creative confidence, learning from failure and optimism. Seeing their students and themselves enhance and ...
Overview. Design thinking is a methodology for creative problem solving. You can use it to inform your own teaching practice, or you can teach it to your students as a framework for real-world projects. The set of resources on this page offer experiences and lessons you can run with your students. This gives educators interested in teaching ...
Design Thinking in Education. Design Thinking has been widely recognized as an alternative way of thinking and methodology for problem solving in Education institutions from K-12 to Grad Schools in educational institutions such as Stanford, MIT, Darden School of Business at Virginia University, Carnegie Mellon and many more.
This study aimed to analyze articles published in the Web of Science database from 2012 to 2021 to examine the educational goals and instructional designs for STEM education. We selected articles based on the following criteria: (a) empirical research; (b) incorporating instructional design and strategies into STEM teaching; (c) including intervention; (d) focusing on K-12 education and on ...
Abstract. Design Thinking has become an established approach in science and the commercial sector so that companies and institutions worldwide are benefiting from this new problem-solving and innovation mindset. At HPI, we have learned over the past 15+ years that design thinkers, whether students or professionals, develop a more thorough ...
Design thinking is an iterative, non-linear process which focuses on a collaboration between designers and users. It brings innovative solutions to life based on how real users think, feel and behave. This human-centered design process consists of five core stages Empathize, Define, Ideate, Prototype and Test.
Through the above course teaching design, we integrate system thinking as the core teaching concept and method throughout the course, achieving good teaching results in course construction and teaching practice. References [1] ... Journal of Higher Education Research, 2023, 46 (03): 26-30.
The C4DLab is running a week-long training program for faculty on Design Thinking and Problem-Based Learning, innovative problem-solving approaches. The training, aptly dubbed "Innovation Training" for revolutionary approach to solving real-world problems, is currently underway in the PhD room of the Department of Computing and Informatics.
The Discipline-Based Science Education Research Center (dB-SERC) has awarded 12 Course Transformation Awards to faculty in natural sciences. Since 2014, dB-SERC has supported natural sciences faculty members in developing projects to transform the way classes are taught by adopting evidence-based teaching practice to improve student learning outcomes. Award recipients receive funds for ...