
Maintenance work is planned from 21:00 BST on Sunday 18th August 2024 to 21:00 BST on Monday 19th August 2024, and on Thursday 29th August 2024 from 11:00 to 12:00 BST.
During this time the performance of our website may be affected - searches may run slowly, some pages may be temporarily unavailable, and you may be unable to log in or to access content. If this happens, please try refreshing your web browser or try waiting two to three minutes before trying again.
We apologise for any inconvenience this might cause and thank you for your patience.

Materials Chemistry Frontiers
Recent advances in organic light-emitting diodes: toward smart lighting and displays.

* Corresponding authors
a Jiangsu Key Laboratory for Carbon-Based Functional Materials & Devices, Institute of Functional Nano & Soft Materials (FUNSOM), Soochow University, Suzhou, Jiangsu, P. R. China E-mail: [email protected] , [email protected]
b School of Physics and Electronics Science, Ministry of Education Nanophotonics & Advanced Instrument Engineering Research Center, East China Normal University, Shanghai, China
c Institute of Organic Optoelectronics (IOO), JITRI, Wujiang, Suzhou 215215, China
Organic light-emitting diodes (OLEDs) have rapidly grown as one of the leading technologies for full-color display panels and eco-friendly lighting sources due to their outstanding features including superior color quality, wide viewing angle, mercury-free manufacture, fascinating flexibility, etc. A variety of materials, device architectures, as well as processing techniques have been investigated for optimizing device performance in order to fulfill the requirements of lighting and display applications. In this review, we first summarize the light emission mechanisms of electroluminescent materials. Then, the designed device architectures aiming at the realization of various light emission mechanisms are reviewed. An overview of recent advances in light extraction strategies is presented since all efficient OLEDs have a multi-thin-film structure, which leads to severe light trapping in devices. In addition, the progress of flexible OLEDs is reviewed from the aspect of flexible transparent electrodes because of their great potential in flexible displays. Most recent breakthroughs of solid-state lighting and displays are briefly addressed as well. A brief perspective on future research is also proposed for pursuing the commercialization of OLEDs.
- This article is part of the themed collection: 2020 Materials Chemistry Frontiers Review-type Articles
Article information
Download citation, permissions.

S. Zou, Y. Shen, F. Xie, J. Chen, Y. Li and J. Tang, Mater. Chem. Front. , 2020, 4 , 788 DOI: 10.1039/C9QM00716D
To request permission to reproduce material from this article, please go to the Copyright Clearance Center request page .
If you are an author contributing to an RSC publication, you do not need to request permission provided correct acknowledgement is given.
If you are the author of this article, you do not need to request permission to reproduce figures and diagrams provided correct acknowledgement is given. If you want to reproduce the whole article in a third-party publication (excluding your thesis/dissertation for which permission is not required) please go to the Copyright Clearance Center request page .
Read more about how to correctly acknowledge RSC content .
Social activity
Search articles by author, advertisements.

- Search this journal
- Search all journals
Article Sections
- LED Light Growth Spectra: The PAR Region
- Spectra Beyond PAR
- Supplementary and Intracanopy Lighting
- Other Considerations for LED Lighting
- LED Lighting: Is There a Price to Pay?
- References Cited
Article Figures
( A ) Wavelengths of the electromagnetic spectrum absorbed by plants and used to drive photosynthesis. Three main regions are identified: ultraviolet, associated with potentially damaging high energy photons of light; photosynthetically active radiation (PAR), the spectra that is absorbed by plant pigments to provide light energy for photosynthesis; and far-red (FR) light, associated with lower-energy photons of light. Key photoreceptors and their associated absorption spectra are also identified, showing the regions of ultraviolet, blue, red, and FR light detection by associated photoreceptors. Cryptochromes and phototropins have a role in blue light sensing—for instance, as used in phototropism or the increase in plant pigments to protect from high light intensity. Phytochromes are responsible for FR and red light sensing—for instance, to regulate plant growth in response to an increase in FR light associated with shade. UVR8 senses ultraviolet light and, much like blue light receptors, is involved in regulating high light to ultraviolet-protective pigments. ( B ) The photon flux density of irradiating sunlight per nanometer wavelength, based on the average for the 48 contiguous U.S. states over a period of 1 year, with ( C ) a close-up look at the visible spectra showing higher light intensities in green, orange, and red wavelengths than those of blue wavelengths, exhibiting a total photosynthetic photon flux density of 1739 μmol·m −2 ·s −1 . The photon flux density was calculated based on the ASTM G-173 reference spectra, accessible at nrel.gov/grid/solar-resource/spectra-am1.5.html.
Example spectra of common light sources for the purpose of plant growth. ( A ) A typical white light-emitting diode (LED) with a color temperature of 5700 K. ( B ) A selection of LEDs for the wavelengths of 450 nm (blue), 520 nm (green), 660 nm (red), and 735 nm (far-red). These are common wavelengths used by many manufacturers; however, many more wavelengths are available. Actual relative intensities will vary between manufacturers, and a uniform maximum intensity is shown here for illustration purposes. ( C ) A fluorescent bulb. ( D ) A high-pressure sodium (HPS) lamp used for supplementary lighting. ( E ) HPI, a type of metal halide light. ( F ) An example LED spectrum, composed of the spectra of panels A and B, demonstrating the flexibility afforded by LED lighting. Spectra for each light source type was measured in-house at the University of Essex, showing the relative photon flux density for each light source, and therefore the light intensity between light sources in this figure are not directly comparable. Calculated red:blue ratios can be found in Supplementary Table 1.
An example protocol outlining the steps for determining the optimal red-to-blue (R:B) ratio for crops. This example protocol can be used for plants that a grower has not grown under light-emitting diode lighting before and serves as a starting point for determining the optimal R:B ratio. In the first step, three base ratios are suggested, with higher proportions of blue ( A ) or red ( C ), or a R:B ratio similar to sunlight ( B ). The base ratio to choose depends on whether, for instance, biomass or secondary metabolites are the primarily factor desired ( D ). The second step is to assess the crop that has been grown under one of the base regimes. Are plants grown with sufficient biomass, compaction, coloration, and desired secondary metabolites? The third step is to adjust the R:B ratio (e.g., for a base R:B of 3, adjust from 3 to 5 for more red, or 3 to 1.5 for more blue). Repeat steps 2 and 3 until the optimal (or close to optimal) R:B ratio is determined.
Some of the major modifications to the light-emitting diode (LED) growth spectra and their resulting effect. The effects listed here are generalized, not exhaustive, and are dependent on species and other environmental factors. In the ‘Spectra’ column, arrow colors refer to spectral region, typically; purple for ultraviolet light (<380 nm), blue for 400–500 nm, green for 500–600 nm, red for 600–700 nm, dark red for 700–750 nm. White arrows refer to the general spectrum a grower has previously chosen for their plants. Arrow sizes represent the approximate quantity of light. For end-of-day (EOD) and night-break treatments, the boxes colored yellow represent light, white boxes represent night, and colored boxes represent a light treatment without any other light source.
Some of the major phytochrome-mediated effects due to red or far-red light. Red light converts the Pr form of phytochrome to the Pfr form, which regulates normal unshaded growth. Far-red light converts the Pfr form of phytochrome to the Pr form, which promotes signaling pathways that promote plant growth and flowering. Upward arrow denotes an increase, whereas a downward arrow denotes a decrease. * An effect that has not been demonstrated in all relevant crops.
Productivity increase (mean of leaf area, leaf number, biomass, height) from changes in light spectral quality for horticultural crops (e.g., spinach, lettuce, rocket) from a control “white” light source to a specific treatment. Control light sources are, for example, high-pressure sodium (HPS), white, or red+blue light-emitting diodes (LEDs). Productivity changes are calculated where some portion of the control light is replaced by the spectrum named. Note that for RB interventions, we assume that 100% of the control (i.e., HPS) lighting is replaced with a new red + blue spectrum LED. For monochromatic treatments, we estimate that 20% (blue), 20% (green), 50% (red), and 12.5% (far-red) of the control spectrum is replaced (see text). Error is standard error of average values as reported in the literature. All data sources can be found in the Supplementary Materials.
Annual electricity cost of light-emitting diodes (LED) use (m −2 ) based on usage implied in research papers (see Supplementary Materials). Red, blue, green, and far-red may be given to replace (part of) baseline R:B or white light (see Fig. 6 ). Costs are based on 18 h·d −1 of 250 μmol·m −2 ·s −1 total PFD, with light treatments as described in Fig. 6 . Electricity price is based on a medium-sized electricity user in the United Kingdom in the first quarter of 2022 including Climate Change Levy, a tax on energy used by nondomestic users; growers in receipt of feed-in tariffs or similar incentives might find ways to reduce input costs. Results assume 2.5 μmol·J −1 efficacy of LED units and 1.75 μmol·J −1 efficacy of high-pressure sodium units ( Kusuma et al. 2020 ), and 6570 h use per annum (i.e., 18-h days), no credit is given for the white light/R:B light replaced.
Comparison of yield increase ( Fig. 6 ) against electricity costs ( Fig. 7 ). Dot color corresponds to light treatments, darker red dot corresponds to far-red light; bright red dot, red light; blue dot, blue light; green dot, green light; blue-red gradient dot, a mixture of both red and blue light. The benefits of using far-red light dominate all other options for marginal cost in this model. However, the link between spectral intervention and cost implication remains complex. The initial cost of moving to LED lighting (Red+Blue) is a considerable barrier to investment notwithstanding ongoing declines in installation cost; nevertheless, LEDs typically offer a decrease in cost in excess of 50% compared with high-pressure sodium ( Nelson and Bugbee 2014 ) and may be attractive in their own right. Given the presence of an existing white or R:B lighting system, augmentation of particular spectra appears attractive. Far-red, blue, and, to a lesser extent, green augmentation offer the potential for attractive increases in yield yet only modest increases in running costs.
- View raw image
- Download Powerpoint Slide
Related Content
- Next Article
LED Lighting: A Grower’s Guide to Light Spectra
Click on author name to view affiliation information
For many growers, established and newcomers, the determination of the optimal light spectrum for growing crops can be challenging and highly dependent on crop species and variety. With the increased popularity of LED lighting, the capability to fine-tune a light spectrum has never been greater. Here, we break down the fundamental roles of the major spectral regions (ultraviolet, blue, green, red, and far-red) and explain the effect on plant growth, yield, and crop quality (i.e., greenness, coloration, flavor) when applied in isolation or combination. The first part of this review examines plant responses to light stimuli and the potential benefits for growers. We also discuss how LED lighting can be used to manipulate plant growth and development to improve crop productivity and/or value. We suggest some basic LED light “recipes” that could be used by growers to deliver specific growth effects and provide an easy-to-use visual reference guide. The second part of this review explores the impact of light treatments on crop productivity. Increased productivity is weighed against the ongoing costs associated with various light treatments, modeled in the context of UK electricity pricing.
Light is an essential resource for all plants, providing the energy necessary for photosynthesis, the process that enables plants to grow. However, light also plays a major role in influencing plant morphology and physiology, which is dependent not just on light intensity but also the spectral quality (color) of light. The effects of intensity and quality on plant performance and morphology are discussed in this review, with emphasis on how light can be used to improve the quality and quantity of crop yield.
Light is typically discussed in terms of light particles, or photons. Photons have specific wavelengths, ranging from the short wavelengths of the electromagnetic spectrum such as gamma and X-rays (<10 nm), to the long wavelengths of radio and microwaves (>1 mm). Visible light ranges between wavelengths of 400 and 700 nm ( Fig. 1A ). The wavelength of a photon is inversely correlated to the energy of that photon, with shorter wavelengths having higher energy, as described by Planck’s equation of E = hc/λ; in which E is energy (Joules), h is the Planck constant (6.62607015 × 10 −34 J·s), c is the speed of light, and λ is wavelength. The approximate relationship between wavelength and visible light as seen by the human eye is illustrated by the corresponding colors in Fig. 1A . When referring to the quantity of incident light on a plant, the photon flux density (PFD) is the most commonly used measurement of light intensity, which refers to the number of photons (µmol) received on a specified area (m 2 ) per second (µmol·m −2 ·s −1 ), with 1 mol of photons consisting of 6.022 × 10 23 photons.

Citation: HortScience 58, 2; 10.21273/HORTSCI16823-22
- Download Figure
- Download figure as PowerPoint slide
In the leaf, light between 400–700 nm ( Fig. 1A ), a region of the electromagnetic spectrum which is referred to as photosynthetically active radiation (PAR), is absorbed by various plant pigments. The total PFD for light within the PAR (400–700 nm) region is referred to as photosynthetic photon flux density (PPFD) and is the measurement used when assessing the impacts of light intensity on plant growth. However, recently there has been considerable debate regarding the definition of PAR, with suggestions that it should be extended to include far-red (FR) wavelengths up to 750 nm ( Zhen et al. 2022 ; Zhen and Bugbee 2020a , 2020b ; Zhen and van Iersel 2017 ). Other measurements commonly used include irradiance, in units of Watt per m 2 (W·m −2 ), which measures the overall power (referred to as radiant flux) received per unit area. The difference between PPFD and irradiance is that PPFD (µmol·m −2 ·s −1 ) measures photons, whereas irradiance (W·m −2 ) measures energy. The two are interconvertible bearing in mind that the energy of a photon depends on its wavelength as per Planck’s equation. Lux is another measurement often provided by lighting manufacturers, which indicates the amount of lumens per square meter. The measure of lumens is weighted to the sensitivity of the human eye to each wavelength. Thus, given the same output of light in terms of irradiance (W·m −2 ), a green light will have a higher lux reading than a blue or red light. Red and blue wavelengths are particularly important to plants, and therefore PPFD or irradiance based units should be employed when referring to light intensity relative to crop performance, and lux should not be used unless a fixed spectrum is used and the relationship between PPFD and lux under that spectrum known.
Absorption of light for photosynthesis is predominantly due to chlorophyll pigments, which have absorbance peaks in red and blue wavelengths, with accessory pigments extending absorption to the other wavelengths in the visible spectrum ( Lichtenthaler and Buschmann 2001 ). Photosynthesis is the process by which light energy (from the sun or electric lamps) is used to convert carbon dioxide (CO 2 ) and water into sugars, which are required for all plant growth and maintenance. Thus, light is clearly crucial in maintaining high rates of photosynthesis and plant growth.
Although light is essential for photosynthetic processes, too much light can be damaging, particularly in conjunction with other environmental stressors. Plants are sessile organisms and are unable to move rapidly in response to changing environmental cues or threats, including excess light energy (i.e., where more light energy is absorbed than can be usefully harnessed for electron transport). They have therefore developed an array of mechanisms to monitor and respond to environmental conditions to ensure survival and reproductive success ( Casal 2013 ; Jenkins 2017 ). Both light intensity and spectral quality are triggers to which plants respond and adjust on both short- and long-term scales. For instance, plants have developed mechanisms to dissipate light energy in excess of that required for photosynthesis. One primary means of dissipating excess excitation energy is through pigments such as carotenoids, which dissipate absorbed light energy as heat (a process known as nonphotochemical quenching). This aids in preventing excess light energy from producing reactive oxygen species (ROS) and free radicals, which can significantly damage proteins, lipids and pigments, and subsequently reduce photosynthetic performance ( Latowski et al. 2011 ; Murchie and Lawson 2013 ; Mullineaux et al. 2018 ; Murchie and Harbinson 2014 ). ROS accumulation is prevented by a battery of antioxidants such as ascorbic acid (vitamin C) and α-tocopherol (vitamin E; Mullineaux et al. 2018 ). Other pigments such as anthocyanins, which give leaves a distinctive purple color, also assist in reducing light absorption by photosynthetic pigments and can act as antioxidants helping to remove ROS ( Chalker-Scott 1999 ; Kovinich et al. 2015 ; Thoma et al. 2020 ; Zheng et al. 2020 ). Many of these antioxidants and pigments are also highly desired by some crop growers and retailers, due to the nutritional and visual attractiveness of these products to consumers.
These photoprotective mechanisms assist in maintaining optimal rates of photosynthesis under periods of high light ( Murchie and Harbinson 2014 ; Murchie and Lawson 2013 ; Murchie and Ruban 2020 ). Thus, light intensity and subsequent photo-protective mechanisms have a major impact on plant growth. Low light intensity can limit plant growth, whereas too much light can be detrimental and damage plants, but additionally the speed of recovery from fluctuations in light intensity through management of nonphotochemical quenching capacity is linked to sustained rates of photosynthesis and yield ( Casal 2012 ; Chavan et al. 2020 ; Kromdijk et al. 2016 ).
Other examples of environmental cues that influence plant development include changes in daylength, as experienced in spring and autumn months, which are major signals that plants use to determine seasons and flowering time ( Johansson and Staiger 2015 ). The spectral quality of light is another key aspect that influences plant growth. Plants are able to detect the presence and intensity (or quantity) of light in specific spectral regions, typically grouped into ultraviolet, blue, red, and FR ( Fig. 1A ), through photoreceptors such as phototropins, cryptochromes, and phytochromes ( Fig. 1A ). Changes in light spectral quality, which, for example, can arise due to shading from other plants, result in the activation and deactivation of signaling pathways, leading to changes to plant growth, morphology, or performance.
Light sources: natural and electrical.
Light from the sun is refracted and absorbed by atmospheric particles, such as water as it passes through Earth’s atmosphere. This absorption affects the spectrum of sunlight that reaches the surface of Earth. A typical surface solar spectrum ( Fig. 1B ) exhibits a large peak corresponding to wavelengths of visible light. The visible spectrum ( Fig. 1C ) has increasingly higher intensities of green, orange, and red (wavelengths from 500 to 700 nm) relative to blue. However, sunlight is not always available or of sufficient intensity for growing certain crops or at particular times of the season. Supplementary lighting, which refers to electric lamps used to supplement sun-lit plants, is often employed by growers in greenhouses and polytunnels when natural light is limited due to low transmission through glass or plastic coverings, cloud cover, shading from nearby structures, and when natural light levels are low (e.g., winter months) ( Chavan et al. 2020 ; Palmer and van Iersel 2020 ). Supplementary lighting is also used to extend the daylength to induce flowering in long day (short night) plants or to maximize flowering by optimizing the daylength for day-neutral plants ( Hidaka et al. 2014 ). In some cases, all a crop’s lighting requirements may be met with electric lamps, a common approach in the increasing use of controlled environment agriculture, which are sometimes referred to as “plant factories”—large warehouses composed of tall stacks of hydroponic systems also referred to as vertical farms ( Kozai et al. 2019 ; Touliatos et al. 2016 ).
Several lighting options are available to growers ( Fig. 2 ), each with different spectral qualities. In addition to ambient sunlight, there is the option of high-pressure sodium (HPS), metal halide (MH), fluorescent or light emitting diode (LED) lights, each with advantages and disadvantages for specific situations, most commonly relating to spectral output. HPS lighting is rich in orange–red wavelengths ( Fig. 2D ) with small amounts of blue and green, whereas MH lights typically have peaks in blue, green, and orange wavelengths ( Fig. 2E ). Most electric lamps emit heat energy along with useful irradiance, which either has to be managed within greenhouse climate control systems or used as part of the heating system ( Bakker et al. 2006 ; Firfiris et al. 2012 ). LED lighting is opening up new avenues for growers, allowing almost complete flexibility in the control of the light spectrum, intensity, and scheduling. Not only do LEDs offer unparalleled control over the lighting spectrum, but the reduced loss of energy to heat output contributes to the lower energy consumption of LED lights, with reported reductions of up to 20% to 35% compared with conventional HPS, greatly reducing energy consumption relative to traditional illumination systems ( Kaukoranta et al. 2017 ; Pattison et al. 2018 ; Särkkä et al. 2017 ). As a result, LEDs have quickly overtaken other light sources as the first choice for many growers, especially given their spectral flexibility and ability to vary intensity. Unlike MH or HPS, LEDs can also turn on and off at will, whereas other types of lighting often requires a substantial (10–15 min) period of warming up and cooling down, and therefore cannot be switched rapidly. Having said this, LEDs do not have as long a history of use for growing plants as other lights, and therefore the relationship between LED spectra and crop performance is relatively unknown and untested. Furthermore, the impact of LEDs on crop growth will differ in crop varieties and growth environments, such as temperature, nutrients, irrigation, and humidity, and therefore it can be difficult to draw a concrete conclusion as to the effects of light on crop yield. However, general trends can be identified and used as a baseline for further fine-tuning. In addition to this, the associated initial costs with transitioning to a LED-based setup means that uptake so far has been limited.

The importance of red and blue light.
Red (600–700 nm) and blue (400–500 nm) wavelengths are the primary wavelengths employed in most LED based lighting systems as they are most strongly absorbed by plant pigments (including chlorophylls) for photosynthesis ( Lichtenthaler and Buschmann 2001 ; McCree 1971 ). Red light is more efficiently used in photosynthesis than blue light ( McCree 1971 ) due to several factors. First, absorbed high-energy blue light is transferred to lower energy chromophores in the photosynthetic reaction center, with the remainder of that energy lost as heat, and thus absorbed blue light delivers the same amount of energy for photochemistry as absorbed red light ( Heldt 2005 ; Mirkovic et al. 2017 ). Second, blue light is absorbed not just by chlorophyll but also other pigments such as carotenoids, which have an absorption spectrum that overlaps with chlorophyll, mainly in the blue spectral region; however, there is low efficiency in the transfer of energy from carotenoids to chlorophyll ( Lichtenthaler and Buschmann 2001 ; Mirkovic et al. 2017 ; Peterman et al. 1997 ; Stamatakis et al. 2014 ). Thus, blue light is associated with the production of compounds to aid in the dissipation of excess absorbed light energy, referred to as secondary metabolites ( Huché-Thélier et al. 2015 ; Thoma et al. 2020 ), which are often associated with increased nutritional value or are contributing factors to the taste and flavor of vegetables and fruits. Additionally, a higher pigment content due to blue light has been shown to improve the recovery of plants exposed to ultraviolet stress ( Hoffmann et al. 2015 ).
However, narrow-band red or blue light is often associated with poor plant performance and growth ( Larsen et al. 2020 ; Ouzounis et al. 2016 ; Trouwborst et al. 2016 ; Zhang et al. 2019 ). Growth under narrow-band red light can give rise to “red light syndrome” in part due to a lack of blue light receptor mediated photomorphogenesis ( Larsen et al. 2020 ), which reduces rates of photosynthesis and photosynthetic capacity ( Hogewoning et al. 2010 ). The addition of blue light to narrow-band red light can return plants to “normal” photosynthetic rates within a matter of days, although morphological changes due to red light syndrome are not always fully recovered ( Trouwborst et al. 2016 ; Wang et al. 2016b ). Narrow-band light can alter plant morphology and improve certain desirable traits—for example, increased fresh and dry weight of shoots ( Johkan et al. 2010 ; Wollaeger and Runkle 2015 ) and increased plant height ( Hirai et al. 2006 ; Johkan et al. 2010 ; Rabara et al. 2017 ; Wollaeger and Runkle 2015 ) have been reported in plants grown under narrow-band red light. Similarly, narrow-band blue light can increase root dry weight and pigment content, which conferred an advantage for transplanted lettuce plants ( Lactuca sativa ; Johkan et al. 2010 ), and, depending on species, increased stem elongation, dry mass, and leaf area ( Hernández and Kubota 2016 ; Hirai et al. 2006 ). For example, elongation under narrow-band blue has been reported for many microgreens, which improves microgreen crop desirability to consumers ( Brazaitytė et al. 2021 ; Ying et al. 2020b ). The general effect of narrow-band light is best illustrated in marigold, an ornamental plant, which produced taller stems and greater or increased internode lengths under narrow-band blue; however, dry weight was higher under narrow-band red light ( Heo et al. 2002 ). However, in the majority of cases, the combination of both red and blue light within a growth spectrum results in improved overall crop growth and better performance than narrow-band red or blue. Therefore, the determination of the optimal ratio between red and blue light (R:B ratio) is one of the most important characteristics of a growth light spectrum. However, the specific ratio of red to blue depends on the crop; the desired outcome for the grower; and balance between growth rates, biomass, compaction, and pigmentation.
Although a standardized light spectrum may not exist, many LED manufacturers typically produce “grow lights” with a spectrum of ∼80% red and 20% blue (R:B of 4) ( Särkkä et al. 2017 ). On the other hand, the light spectrum for standard (cool) white 5700 K LEDs, which is a common white LED used for growing plants, have a R:B ratio of 0.66 (40% red, 60% blue). Therefore, the first question a grower transitioning to LED lighting is likely to ask is, “What ratio of red and blue light should I be using?”
A summary of responses by common crops to different R:B ratios is shown in Tables 1 and 2 , with the effects separated depending on whether the light source is sole-source (i.e., provided entirely artificially by electric lamps, without sunlight; Table 1 ) or supplementary (Table 2). For sole source red + blue LED lighting, a clear trend emerges for increased pigment content with increased blue—namely, anthocyanins and chlorophyll content ( Table 1 ), although there are some cases in which too much blue was associated with decreased chlorophyll content ( Naznin et al. 2019 ; Pennisi et al. 2019 ; Son and Oh 2015 ). Likewise, although blue was associated with increased secondary metabolites ( Naznin et al. 2019 ; Son and Oh 2015 ; Ying et al. 2020a ), a higher red:blue ratio has also been shown to increase certain secondary metabolites, for example, flavanols in basil ( Ocimum basilicum ; Pennisi et al. 2019 ). Morphologically, increasing the fraction of blue light leads to more compact plants, as shown by decreases in plant height and leaf area, and thus a resulting decrease in fresh/dry weight and thicker leaves ( Table 1 ).
The responses of crops grown under controlled environments with different combinations of red and blue light. When an exception is mentioned, the specific treatment goes against the overall trend, and in some cases may have the highest or lowest values.

The responses of crops grown with different combinations of supplementary red and blue light.

Under supplementary lighting, the story remains the same ( Table 2 ) in that higher fractions of blue light result in higher photosynthetic capacity and rates. As with sole source lighting, the increasingly compact size of plants grown under high supplementary blue fractions are also prone to lower biomass. Compaction in plants with increasing fractions of blue are mostly likely the results of poorer light interception (due to smaller canopies), which ultimately lowers biomass ( Izzo et al. 2021 ; Kaiser et al. 2019a ). Therefore, it has been suggested that the addition of supplementary blue light is only optimal up to a certain amount ( Kaiser et al. 2019a ).
While these general trends hold true and reliably allow for R:B ratios to be determined to best suit desired crop performance and characteristics, there are some additional factors to consider. For instance, in fruit-bearing crops such as strawberry ( Fragaria × ananassa ) and sweet pepper ( Capsicum annuum ), higher rates of photosynthesis were observed under lower R:B (higher blue), however higher fruit yield was found under higher R:B (higher red) ( Naznin et al. 2019 ; Piovene et al. 2015 ). The decrease in fruit yield despite high photosynthesis in plants grown in a high compared with low R:B ratio may be due to greater partitioning of assimilates to leaves instead of the fruits, which can also occur in crops grown under high light intensity ( Trouwborst et al. 2011 ). It has also been proposed that a higher fraction of blue light causes the plant to behave as if it is under a higher light intensity ( Lichtenthaler et al. 1980 ), supporting higher chlorophyll content and photosynthetic capacity ( Table 1 ), indicating that plants perceive light intensity as blue light ( Matsuda et al. 2007 ; Zhang et al. 2019 ).
In summary, it is clear there are discrepancies between studies, with differing optimal R:B ratios observed for the same species. The variation seen between studies is likely due to the variations in experimental conditions such as PPFD, addition or omission of wavelengths such as green and FR, nutrient availability, temperature, and other environmental factors, as well as species and choice of cultivar. Although LED lighting offers complete spectral freedom, this comes at the expense of spectral consistency between growers and setups, which can make it difficult to unravel the effects of differing spectral conditions on crop performance. Despite this, there is an overall trend for greater biomass with increasing fractions of red light, whereas higher fractions of blue tend to increase photosynthetic capacity, which is beneficial under higher light intensities (e.g., under sunlight) ( Hogewoning et al. 2010 ; Kaiser et al. 2019a; Kang et al. 2021 ), pigment content, and secondary metabolite synthesis ( Tables 1 and 2 ). Although these general trends are worth adhering to (and a basic protocol for determining the optimal R:B ratio is provided in Fig. 3 ), it is also worth keeping in mind that the response to increasing red:blue ratios can vary based on species, cultivar, and environmental conditions.

Green light.
Altough often overlooked, green light is also important for plant performance ( Fig. 4 ). Typically defined as the region of light wavelengths from 500 up to 600 nm ( Fig. 1A ), green light is able to penetrate deeper into the leaf, driving photosynthesis in lower layers that may be limited by the absorption of red and blue light by pigments in the upper leaf layers ( Smith et al. 2017 ; Terashima et al. 2009 ). Enhanced green light has been associated with increased plant growth in lettuce and increased yield in tomato ( Solanum lycopersicum ; Kaiser et al. 2019b ; Kim et al. 2004 ). A greater proportion of green may be beneficial at higher PPFD values (i.e., 1000 µmol·m −2 ·s −1 ) because a proportion of the absorbed blue and red light in the upper leaf layers at high light intensities will be dissipated as heat, thus reducing photosynthetic efficiency, whereas the lower absorbance of green in the upper layers allows greater penetration to the lower layers where it can be absorbed more efficiently, driving photosynthetic processes ( Liu and van Iersel 2021 ). Green light also has other roles in regulating plant performance, for instance by reducing the accumulation of nitrates by increasing nitrate assimilation ( Bian et al. 2018 ).

Green light has also been reported to counteract many processes initiated by red or blue irradiance ( Folta and Maruhnich 2007 ). For example, green light reversed blue light–induced stomatal opening, resulting in closure of stomata ( Frechilla et al. 2000 ; Matthews et al. 2020 ), and this mechanism has demonstrated potential in improving plant water use and drought tolerance in tomato ( Bian et al. 2019 ). Finally, too much green light in comparison with blue light can have consequences on plant morphology (Wang and Folta 2013), triggering shade avoidance responses in basil, resulting in rapid growth at the expense of pigment content and compactness ( Schenkels et al. 2020 ).
Ultraviolet.
As mentioned in the opening section, photons of light with shorter wavelengths have higher energy. Therefore, blue and ultraviolet light have the highest energy out of all the wavelengths considered in this review ( Fig. 1 ). Blue light is used in photosynthesis, but too much blue light triggers plant defense mechanisms to protect the plant significant damage to proteins and pigments ( Latowski et al. 2011 ; Mullineaux et al. 2018 ; Murchie and Harbinson 2014 ).
Although ultraviolet light can be even more damaging owing to higher energy than blue and is not used directly in photosynthesis, it can also be beneficial to growers. Many plants respond to ultraviolet (and similarly to increasing intensities of blue light) by producing secondary metabolites—compounds not directly involved in normal growth and development, but which aid in plant defenses to external threats such as high light stress, disease, and herbivory ( Huché-thélier et al. 2015 ). For instance, chemical growth regulators are often applied to cucumber ( Cucumis sativus ) to modify plant growth and fruit yield ( Tantasawat et al. 2015 ), with one common use of growth regulators being to reduce plant size without affecting overall fruit yield. Applying ultraviolet-B (280–315 nm) light to cucumber as an alternative to these growth regulators demonstrated that while at low doses of ultraviolet-B no major impact on fruit yields were observed, more compact plants with higher levels of antioxidants, and phenolic and flavonoid content were found ( Qian et al. 2020 ), although too high a dose of ultraviolet-B can also negatively impact yield ( Qian et al. 2020 ; Topcu et al. 2018 ). These photoprotective benefits from ultraviolet exposure, due to increased secondary metabolites, have been reported to improve performance under dynamic environments such as those experienced outside in the field. For example, in a study in which lettuce seedlings were grown with the addition of ultraviolet-B, performance and yield was greatly improved after transplanting to a field environment ( Wargent et al. 2011 ).
Interestingly, when ultraviolet-A light was used as a direct replacement for blue light, tomato growth was increased due to several morphological adaptions, including greater leaf area and steeper leaf angles, which in turn improved light interception and led to improvements in biomass ( Zhang et al. 2020 ). However, such ultraviolet-A replacement also decreased secondary metabolites production and resulted in lower photosynthetic capacity ( Zhang et al. 2020 ), in contrast to what usually occurs when ultraviolet is added. This contradiction is most likely due to the absence of blue light eliciting a low-light ( Hogewoning et al. 2010 ) or shade avoidance response, which signals the plant to focus on physical growth to escape the shade of neighboring vegetation, rather than an effect of ultraviolet ( Keller et al. 2011 ; Pedmale et al. 2016 ; Zhang et al. 2020 ). This suggests that there is still plenty to explore with more unorthodox combinations of wavelengths, such as a red and ultraviolet light based spectra.
Far-red light.
FR wavelengths (700 to ∼900 nm) are known to be important for photosynthesis through the preferential absorption of these wavelengths by PSI, which drives higher electron flow through PSI, and, as a result, improves electron transfer through the entire electron transport chain, which would otherwise be a bottleneck ( Pettai et al. 2005 ; Zhen and Bugbee 2020a , 2020b ; Zhen and van Iersel 2017 ; Zhen et al. 2022 ); therefore, FR up to 750 nm should be considered in the definition of PAR. However, there are both photosynthetic and photomorphogenic effects of FR that need to be weighed when considering the addition of this spectrum to a lighting recipe ( Fig. 4 ), and this is also discussed in the economic analysis toward the end of this article.
Shade perception and the shade avoidance response.
FR light is not readily absorbed by plant pigments and the majority is reflected or transmitted. Light below the top of the canopy (i.e., in shade) is therefore enriched in FR light and lower in PAR, affecting the ratio of red to FR (R:FR). Environments enriched in FR can trigger a shade avoidance response via the phytochrome photoreceptors, which sense the relative quantities of red and FR light ( Legris et al. 2019 ; Leivar and Quail 2011 ). FR rich environments thus lead to morphological and developmental changes to plant growth that aid the plant in seeking unshaded light ( Fig. 5 ), commonly by increasing internode, stem, petiole, and leaf length; earlier flowering ( Casal 2012 ; Casal et al. 2014 ); increased hyponasty, in which leaves grow vertically rather than horizontally to locate red-rich light; (Polko et al. 2011); and reductions in leaf number and branching ( Wang et al. 2013 ). The effects of FR are also dependent on other wavelengths, with more-pronounced shade avoidance responses under either low light intensity or a high B:R ratio ( Meng and Runkle 2019 ). Shade avoidance responses may also depend on the blue-to-green ratio, mostly via the increase in green light ( Meng et al. 2019 ; Sellaro et al. 2010 ; Wang and Folta 2013; Zhang et al. 2011 ). Also, shade avoidance can be initiated by the upward reflection of FR rich light from vegetation and soil below the canopy ( Green-Tracewicz et al. 2011 ), which may have implications for intercanopy supplementary lighting and the effects of greenhouse floor reflection if FR is added to an overhead lighting spectrum.

FR illumination can also affect disease responses. Whereas red light improved disease resistance in tomato, cucumber, broad bean ( Vicia faba ), watermelon ( Citrullus lanatus ), and roses ( Rosa × hybrida ) through the regulation of plant defense hormones such as salicylic acid ( Nagendran and Lee 2015 ; Rahman et al. 2003 ; Suthaparan et al. 2010 ; Wang et al. 2010 ; Yang et al. 2015 , 2018 ), FR light reduced resistance to Botrytis cineria in tomato and powdery mildew in cucumber, indicating that the balance of red to FR is also important for optimizing resistance to plant disease ( Courbier et al. 2021 ; Ji et al. 2019 ; Shibuya et al. 2011 ; Wang et al. 2010 ).
Due to the energy required for rapid growth, the shade avoidance response also lowers leaf chlorophyll content ( Meng and Runkle 2019 ; Smith and Whitelam 1997 ). Although less chlorophyll may not affect photosynthetic output ( Heraut-Bron et al. 1999 ; Kalaitzoglou et al. 2019 ), consumers prefer deep greenness in leafy crops such as rocket/arugula (common names for both Eruca sativa and Diplotaxis tenuifolia ; Siomos and Koukounaras 2007 ). On the other hand, a high R:FR ratio will elicit an inverse shade avoidance response, increasing chlorophyll content while decreasing height and internode length ( McMahon et al. 2019 ).
FR treatments for manipulating crop development and yield.
As a result of both its photosynthetic activity and shade avoidance response, the addition of FR can increase biomass ( Yang et al. 2020 ). FR has been associated with increased shoot weight and leaf expansion in seedlings and harvest yields of basil and lettuce ( Jin et al. 2021 ; Meng and Runkle 2019 ). For fruiting plants, while FR increased the partitioning of dry weight into the stem and thus led to taller plants in both tomato and pepper ( C. annuum ), a higher fruit mass was also reported in tomato ( Brown et al. 1995 ; Kalaitzoglou et al. 2019 ; Kim et al. 2019a ; Lanoue et al. 2022 ). Although FR showed little effect on pepper fruit yield, it did induce longer internode length, which may prevent deformation of fruit shape ( Lanoue et al. 2022 ). FR also interacts with the daily light integral (DLI), influencing the induction of flowering. Plants grown at a low DLI with FR exhibit more rapid flowering than treatments without FR, whereas at a higher DLI, flowering time is more rapid with or without FR light ( Garrett Owen et al. 2018 ). However, in one study, blue light attenuated FR associated growth, alleviating morphological changes such as increased plant height, but did not affect flowering time ( Park and Runkle 2019 ).
The application of red or FR as night-breaks can trigger a phytochrome response in plants, in which narrow-band red or FR light leads to the conversion of phytochrome to either the Pfr or Pr form of phytochrome and subsequent downstream signaling ( Fig. 5 ) and thus has been shown to delay or trigger flowering ( Borthwick et al. 1952 ; Hendricks and Borthwick 1967 ). In short day (long night) plants, the application of a FR flash during the night increases the length of the night (due to the phytochrome being pushed toward the Pr form), whereas the opposite is required for long day (short night) plants, in which a flash of red during the night will stimulate flowering (phytochrome is trigger toward the Pfr form). These manipulations can be reversed by the application of the opposite flash ( Borthwick et al. 1952 ; Hendricks and Borthwick 1967 ). For example, if the nighttime break of a FR flash is followed by a red flash in the short day plant, flowering will not occur (as the plant will still experience a short night as the phytochrome will be mostly in the Pfr form). Night-breaks of less than a few μmol·m −2 ·s −1 of light have been used to initiate earlier flowering and increasing fruit yield in tomato ( Cao et al. 2016 ). The effectiveness of night-breaks for manipulating growth may depend on varieties, as it has been shown that early bolting varieties of spinach are more sensitive to night-breaks than late-bolting varieties ( Hamamoto et al. 2004 ).
There are indications that daytime grow light spectra may also affect the sensitivity of plants to night-break light treatments ( Higuchi et al. 2012 ). Interestingly, the intensity of FR can complicate the phytochrome driven responses because Pr forms of phytochrome can absorb a small amount of FR light, which is magnified at higher intensities ( Kusuma et al. 2021 ).
Similar to night-breaks, “end of day” (EOD) red or FR treatments applied at the end of the photoperiod when growth lights are usually turned off trigger a phytochrome response in plants, which could provide a promising alternative to day-long application of FR spectra. EOD treatments aim to elicit a response similar to a full-day FR treatment but without the associated energy cost or full shade avoidance response, although the boost to photosynthesis as seen when FR is mixed in with the growth spectrum is not observed ( Ilias and Rajapakse 2005 ; Kalaitzoglou et al. 2019 ; Zhen and Bugbee 2020a). For instance, an EOD red or FR treatment can either elicit (FR) or halt (red) the shade avoidance response and subsequently influence plant height of tomato and poinsettia ( Euphorbia pulcherrima ; Cao et al. 2016 ; Islam et al. 2014 ). This EOD technique has applications in grafting, with EOD FR used to promote stem expansion in tomato without loss of leaf area ( Chia and Kubota 2010 ) or EOD red to prevent excessive stem elongation in ornamentals ( Islam et al. 2014 ). Another use of EOD FR light is to decrease the length of time required before the onset of flowering in strawberry, although this can depend on FR dose and daylength ( Zahedi and Sarikhani 2016 ).
Currently the majority of LED lighting in glasshouses does not include FR. The omission of FR can lead to the inverse shade avoidance response described earlier; more compact plants have lower biomass, attributed to a reduced leaf area and subsequent low light interception and to an absent photosynthetic effect ( Kalaitzoglou et al. 2019 ; Zhen and Bugbee 2020a). A certain amount of FR (the amount is dependent on species and overall lighting spectra) appears to be required to generate desirable traits (e.g., photosynthesis boost, biomass, and larger leaf area), but not too much to reduce chlorophyll content, promote excessive elongation, or alter flowering time. The intensity and balance of red and FR should therefore be fine-tuned to manage the potential gain or loss in productivity, flowering time and fruit yield, stem elongation, and other effects on plant morphology. Thus, FR and red treatments are powerful tools to manipulate crop performance.
Supplementary lighting.
The aim of supplementary lighting is to increase light intensity on lower intensity or cloudy days and/or to extend the photoperiod during autumn, spring, and winter months, or even year-round whenever the photoperiod needs to be extended. Supplementary lighting can also be used to modify the light environment by altering its spectral composition, such as alteration of the R:B ratio, against a background of sunlight. Supplementary lighting for greenhouses traditionally uses HPS lamps, which are enriched in red and orange wavelengths. However, LED lighting is quickly gaining favor with growers due to lower running costs and increased flexibility, as well as the ability to customize spectral output. Additionally, supplementary LED lighting has also been shown to promote higher biomass production and more partitioning to reproductive organs than HPS lamps. Plants grown under HPS have higher leaf temperatures due to the greater heat output of HPS lamps and thus, in addition to higher transpiration rates, may exhibit morphological changes to improve heat dissipation, such as smaller leaves to improve heat loss via convection and conduction ( Chaves et al. 2002 ; Kim et al. 2019a ; Wang et al. 2019 ). Supplementary lighting of all types (i.e., LED, HPS) has been shown to improve fruit yield in tomato ( Gómez et al. 2013 ) and phytochemical content in strawberry ( Choi et al. 2015 ). Likewise, in leafy crops supplementary lighting showed an increased length and yield of pea shoots ( Pisum sativum ; Kong et al. 2019 ) and improved flavor, nutrient content, and yield of pak choi ( Brassica campestris ssp. chinensis var. communis ; Zheng et al. 2018 ).
Intracanopy LED lighting.
Although traditionally set up this way, supplementary light does not have to be exclusively above the canopy and face downward. Within-canopy supplementary lighting has received growing interest and refers to the inclusion of LED strips within the canopy (intracanopy) to increase photosynthesis throughout the plant. This technique is being increasingly used by growers to increase productivity and yield of tall crops such as pepper (Jokinen et al. 2012) and tomato, as well enhancing flavor ( Gómez et al. 2013 ; Kim et al. 2019b ; Tewolde et al. 2018 ). Intracanopy lighting has also been used to increase biomass and yield in crops such as cowpea ( Vigna unguiculata ssp. unguiculata ; Frantz et al. 2000 ) and cucumber ( Pettersen et al. 2010 ). This technique benefits from the advancements of LEDs thanks to the cooler temperature of LEDs over other light sources ( Gómez and Mitchell 2016a )
Fluctuating light and shade-flecks due to shading from the upper canopy have been associated with reduced growth ( Kaiser et al. 2018 ; Vialet-Chabrand et al. 2017 ); however, the addition of intracanopy lighting can improve light distribution throughout the canopy ( Frantz et al. 2000 ; Pettersen et al. 2010 ), increasing photosynthesis in lower leaves that would otherwise present a significant metabolic burden for the plant ( Frantz et al. 2000 ). It should be noted that any increases in photosynthesis by intracanopy lighting remain sensitive to the choice of light spectrum ( Murakami et al. 2013 ), and fine-tuning of light spectra may affect rates of photosynthesis more than morphology and fruit yield ( Gómez and Mitchell 2016b ). Although intracanopy lighting is another tool for light spectrum-based manipulation of plant morphology and performance, we note that evidence of impact remains ambiguous. Intracanopy lighting in cucumber was less effective than overhead supplementary lights in improving yields; leaf curling and a greater partitioning of dry matter to leaves rather than fruit adversely affected productivity ( Trouwborst et al. 2011 ). Therefore, a combination of overhead and intracanopy light may be optimal ( Särkkä et al. 2017 ).
Light intensity.
Light intensity plays a pivotal role in determining plant growth, with too high a light intensity having consequences for crop quality through the initiation of plant defense mechanisms and morphological changes to reduce high light induced stress ( Demmig-Adams and Adams 1992 ; Larsen et al. 2020 ; Mullineaux et al. 2018 ). Some of the effects of excessive light include decreased chlorophyll content, photodamage, damaged leaves and fruits, suboptimal growth, and reduced yield ( Ferrante and Mariani 2018 ; Nguyen et al. 2019 ). During winter months, both photoperiod and light intensity are especially crucial, as the low winter sun results in a reduction in overall light intensity throughout the course of the shorter day, which can affect overall growth rates and flowering ( Johansson and Staiger 2015 ).
The definition of “high” light intensity is dependent on the species and environment, with some plants having highest yield at light intensities as low as 90 μmol·m −2 ·s −1 (e.g., the medicinal herb Anoectochilus formosanus ; Ma et al. 2010 ). However, pushing light intensity toward the upper limit of a crop’s known range is important for secondary metabolite production, which is often produced in response to high light to aid in the dissipation of excess absorbed light energy and improves taste and nutritional content in many crops ( Ma et al. 2010 ; Thoma et al. 2020 ; Zou et al. 2020 ). Lettuce grown under higher levels of electric lamp lighting is susceptible to tipburn due to calcium limitation in fast-growing leaves ( Sago 2016 ). The mechanism of tipburn onset has been associated with rapid plant growth driven by higher light intensities and long daylengths, with that rapid growth and cell expansion resulting in lower calcium concentration in the inner leaf and subsequent development of tipburn ( Sago 2016 ). Therefore, if tipburn is prevalent, it may be suitable to provide localized supply of air to improve transpiration rates and thus nutrient uptake and flow ( Ahmed et al. 2020 ; Frantz et al. 2004 ; Goto and Takakura 1992 ). Other solutions, such as selection of tipburn resistant varieties ( Birlanga et al. 2021 ) or a reduction in temperature to slow growth ( Choi et al. 2000 ), may also prevent onset.
The selection of a light intensity and DLI is a key choice that a grower has to make. Several models, such as for hydroponic salads ( Walters and Lopez 2021 ) and leafy microgreens ( Jones-Baumgardt et al. 2020 ), have thus been produced that attempt to predict the impact of light intensity on growth parameters such as fresh and dry mass, plant height, and photosynthetic performance.
Photoperiod.
Photoperiod refers to the period of light that a plant experiences. Many growers have to determine the optimal daylength under which to grow their crops, with longer photoperiods generally corresponding to increased growth rates ( Adams and Langton 2005 ) due to a higher integrated dose of light or DLI. The response to photoperiod varies across species, with both increases and decreases in leaf area possible ( Adams and Langton 2005 ). However, as a general rule, many crops are typically grown under a long daylength, usually 16 h ( Pennisi et al. 2020 ) because this generally corresponds to the longer daylengths during summer months; however, daylengths used can range from anywhere between 12 to 22 h.
It has been suggested that a longer photoperiod at a lower light intensity (“Long/Low”) may be more photosynthetically efficient than a short photoperiod at a high light intensity (“Short/High”), at the same daily light integral ( Elkins and van Iersel, 2020 ). Plants grown under a Long/Low treatment showed increased biomass and higher chlorophyll content despite lower photosynthetic rates, although it should be noted that a number of crops, such as lettuce and chicory ( Cichorium intybus ), may be suboptimal at daylengths of 20 h or more, whereas others such as spinach ( Spinacia oleracea ) are prone to bolting above daylengths of 14 to 16 h ( Adams and Langton 2005 ; Chun et al. 2001 ; Elkins and van Iersel 2020 ; Palmer and van Iersel 2020 ; Pennisi et al. 2020 ; Weaver and van Iersel 2020 ). The mechanism behind the “Long/Low” is due to the lower light intensity improving the efficiency of photosynthesis ( Weaver and van Iersel 2020 ), with less absorbed light energy dissipated as heat. The importance of photoperiod is especially apparent in flowering crops, in which flowering is initiated when the photoperiod reaches a critical daylength. Short day plants flower when the photoperiod is short (and night is long), whereas long day plants flower when the photoperiod is long (night is short).
Elsewhere, continuous light has been proposed as a technique to increase plant biomass and yield either due to higher cumulative rates of photosynthesis or by distributing the light dose across the photoperiod and increasing photosynthetic efficiency ( Lefsrud et al. 2006 ; Proietti et al. 2021 ; Shibaeva et al. 2022 ). However, the effect of continuous lighting can, depending on species, be detrimental to growth and yield through reduced performance—for instance, due to reduced pigment content ( Lefsrud et al. 2006 ) or to stress or injury ( Gaudreau et al. 1994 ; Murage and Masuda 1997 ; Van Gestel et al. 2005 ). Fruiting vegetables such as pepper can be vulnerable to injury from continuous lighting ( Demers and Gosselin 1999 ), although cucumber has been shown to support growth under continuous lighting ( Lanoue et al. 2021 ). This is especially the case for tomato, which is susceptible to damage when grown under continuous lighting ( Demers et al. 1998 ). However, there are genes that confer tolerance to continuous lighting, allowing for cultivars to be bred specifically for this purpose (Velez-Ramirez et al. 2014, 2015). Although the longer days may translate into marginally higher yields, they may not necessarily offset the increased running costs associated with that daylength, as indicated by the significantly lower energy use efficiency observed with increasing photoperiod ( Pennisi et al. 2020 ). Alternating between 12 h of narrow-band red and 12 h of narrow-band blue light over a 24-h period has been used to improve yields and harvest quality in lettuce ( Ohtake et al. 2018 ), and in tomato, such growth conditions had no adverse effects ( Lanoue et al. 2019 ). Similarly, the addition of green light to the spectrum in a continuous lighting regime has been identified as a mechanism to reduce nitrate accumulation in hydroponically grown lettuce ( Bian et al. 2018 ).
Pre- and postharvest light treatments.
Another use of light treatments is to improve the quality of harvested crop by applying specific light treatments during the days before or after harvest. Preharvest regimes can be used to manipulate plants during growth; for example, increasing the proportion of blue light in the days before harvest in lettuce and other leafy greens (with the growth spectra before this tuned toward promoting biomass) resulted in increased production of secondary metabolites and improved appearance and flavor. A similar approach, using blue or red light to increase light intensity, promoted fruit ripening and improved quality of tomatoes and certain leafy greens ( Bliznikas et al. 2012 ; Ngcobo et al. 2020 ; Nicole et al. 2016 ). Likewise, preharvest illumination by FR has been used to improve postharvest cold tolerance of tomato fruit, due to higher synthesis of the plant hormones abscisic acid and jasmonic acid as a result of a low R:FR ratio, thus improving fruit quality during storage ( Affandi et al. 2020 ; Wang et al. 2016a ). Similarly, an application of a 4-day ultraviolet-B treatment before harvest maintained the nutritional quality of basil during storage by increasing the polyphenol and antioxidant content ( Nascimento et al. 2020 ).
When the supply of nitrogen is in excess of what the plant requires, which often occurs when fertilizer (including hydroponic nutrient solutions) is used, an accumulation of nitrates in plants can occur ( Anjana and Iqbal 2003 ; Maynard et al. 1976 ). Excess nitrates can pose serious hazards to human health, and nitrate content is therefore regulated (e.g., by the EU; European Food Safety Authority 2008 ). This is especially concerning for leafy vegetables, in which the whole plant is consumed ( Anjana and Iqbal 2003 ). New LED approaches to prevent nitrate accumulation from reaching high levels have been developed in recent years; for instance, the application of red light spectra, 5 d of continuous lighting before harvest, or 48 h of continuous red-rich lighting have all been shown to decrease nitrate levels in various leafy vegetables, with some varieties of rocket also showing an increase in the secondary metabolite glucosinolate, which is a key flavor compound ( Nicole et al. 2016 ; Signore et al. 2020 ; Wanlai et al. 2013 ).
Interestingly, treatments can also be applied after a crop has been harvested, known as postharvest treatments. The aim of applying postharvest treatments is to maintain photosynthetic activity in harvested crop and delay deterioration, thereby increasing shelf life and quality. Approaches include applying low levels of white light, which maintained chlorophyll, antioxidant, and flavonoid content and delayed senescence (Hasperué et al. 2016). Pulses of low light have also delayed senescence in harvested broccoli, maintaining quality ( Favre et al. 2018 ). This area of research promises to reduce food waste in the supply chain and increase the value of crops sold and is likely to become a ubiquitous intervention in modern food production systems.
Temperature interactions with growth light conditions.
When determining the optimal growth light intensity, one key consideration is the temperature of the growth environment. Higher temperatures increase reaction rates for photosynthetic enzymes ( Berry and Bjorkman 1980 ), although this is only true up to a point; too high a temperature can instead damage plants and reduce photosynthetic rates ( Berry and Bjorkman 1980 ; Lu et al. 2017 ; Matthews and Lawson 2019 ; Stevens et al. 2021 ). Temperature remains important for tracking crop growth and developmental stages: “growing degree days” is calculated based entirely on daily temperature and is a key metric used to track and predict crop growth ( McMaster and Wilhelm 1997 ; Yang et al. 1995 ). The optimal temperature range, as well the maximum and minimum temperatures for some common crops, were listed by Ferrante and Mariani (2018 ) and can be used to determine the ideal temperature of a growth environment for a specific crop.
A study by Zhou et al. (2019 ) examined the relationship between light intensity and temperature and suggested that lettuce grown at lower temperatures (i.e., 15 °C day/11 °C night) were better suited for lower light intensities (i.e., 350 to 500 μmol·m −2 ·s −1 total, or a supplementary light of 350 μmol·m −2 ·s −1 during winter months). Likewise, increasing temperatures (35 °C day/25 °C night) were suggested to be paired with higher light intensities (up to 500–600 μmol·m −2 ·s −1 ), as experienced by field crops from late spring to early autumn. This approach would be especially useful for balancing heating and cooling requirements with light intensity—for instance, in greenhouses or plant factory installations—to ensure that energy use is not excessive due to an incorrect balance between temperature and lighting.
The previous sections of this article have outlined the effects of different light spectra on plant responses and plant morphology in the context of horticulture, yield, and quality ( Fig. 4 ). The translation of the basic research into implementable outputs for growers is more difficult to compare, with relatively few investigations into the costs of implementing lighting solutions compared with their benefits in terms of increased productivity or quality ( Nelson and Bugbee 2014 ; Pattison et al. 2018 ).
As we have emphasized in this review, it can be difficult to compare findings and outcomes in the literature owing to variability in daylength, light intensity, species, and controls between experiments. However, what has received less attention is the variety of choices available and the subsequent economic performance. LEDs have higher initial costs compared with more traditional lighting systems such as HPS; however, the costs of LEDs have recently decreased and are expected to continue to decrease and at a faster rate than other light sources ( Kusuma et al. 2020 ; Nelson and Bugbee 2014 ). Compared with supplementary HPS, one study reported that replacing HPSs with LED lights resulted in a 75% reduction in lighting costs per fruit grown ( Gómez et al. 2013 ). Furthermore, additional cost savings can be made by dynamically regulating supplementary light intensity, based on forecasted solar light levels and electricity price for the day ahead, and this has been shown to have no negative effects on plant growth or visual quality while reducing overall greenhouse costs ( Sørensen et al. 2020 ).
The economies of lighting are crop dependent. Leafy salads, which are not always considered high value, are cost-effective to produce because input light energy is converted into vegetative biomass, most of which is harvested and sold ( Pattison et al. 2018 ). However, only a fraction of input light energy is converted into harvestable fruit in a range of crops such as tomato and other vegetables ( Pattison et al. 2018 ).
Here, we review the literature, to compare electricity costs associated with different light treatments to their final effect on crop yield, given that electricity is one of the largest variable cost a grower is likely to face ( Graamans et al. 2018 ) and is predicted to increase greatly over the coming years. Other qualities that may be desirable to growers that increase value without increasing yield, such as coloration, freshness, taste, and flavor, will not be included in this comparison for simplicity; however, the importance of these elements should not be forgotten. Therefore, optimizing for both yield and quality may involve trade-offs.
The literature reports a wide range of growth and yield metrics for the effectiveness of light treatments, including height, leaf area, leaf number, shoot length, the fresh and dry weight of shoots and total dry weight. Most of these metrics have been collected from studies on crops such as spinach, rocket and lettuce (see Supplementary Materials). We have only included studies that used light interventions in which light treatments replaced some or all of the control lighting (i.e., white or R:B LED, HPS control) with the relevant narrow-band spectrum (i.e., red, blue, green, FR; Fig. 6 ).

To compare the associated running costs of each of these treatments, we used the 2022 first quarter UK electricity price (Department for Business, Energy & Industrial Strategy, 2022). A number of assumptions were made, based on what is typically reported in the literature: for R+B interventions, we assumed 100% of the preexisting “white lights” (which includes fluorescent, HPS) was replaced with red and blue LEDs. For narrow-band LED treatments, we assumed 50% of the pre-existing “white lights” (which includes LED, fluorescent, HPS) was replaced with red LEDs, 20% of the preexisting “white lights” replaced with green; 20% replaced with blue; and 12.5% replaced with FR LEDs. We considered only the ongoing cost of the replacement light and based our assumptions on a constant total output of 250 μmol·m −2 ·s −1 for a 18-h photoperiod with an LED efficacy of 2.5 μmol·J −1 ( Fig. 7 ).

The result of this literature review highlights two treatments that stand out for their potential to increase crop yield ( Fig. 6 ), compared with running costs ( Figs. 7 and 8 ). First, FR light, which has traditionally fallen outside the definition of PAR ( Zhen et al. 2021 ), shows the greatest increase in plant productivity relative to electricity costs ( Fig. 8 ). However, for leafy crops such as spinach, the caveat is that flowering time may be shortened depending on treatment (FR added to growth spectra, EOD FR, etc.). Second, the replacement of HPS lights with a combination of blue, red+blue, or red LEDs alone ( Fig. 6 ) shows considerable promise, with annual red+blue LED electricity costs of 144 USD·m −2 compared with HPS electricity costs of 210 USD·m −2 ( Fig. 7 ).

Considerations and possible recommendations.
The major barrier to initial investment into LED lighting remains the high investment cost of replacing existing HPS or fluorescent lighting with narrow-band LEDs. LED lighting is associated with reductions in electricity use due to reduced heat loss and thus offers considerable efficiency gains compared with HPS systems ( Nelson and Bugbee, 2014 ), which, combined with trending increases in electricity prices (Department for Business, Energy & Industrial Strategy 2022; Eurostat 2022 ), may offset the initial investment cost of transitioning to LEDs. However, the reduction of heat loss from LED lights is often associated with greater energy use by greenhouse heating systems to compensate for the lack of heat emitted from lighting, especially during winter months. Despite these considerations, using LEDs still reduces overall energy use within a greenhouse compared with HPS ( Katzin et al. 2021 ; Kaukoranta et al. 2017 ).
Adding a FR spectrum to the existing white or red:blue spectrum offers the most attractive yield uplift; however, this does not account for any changes to the quality of crop, such as chlorophyll and other pigment content and other aspects of morphology ( Fig. 4 ). Adding blue light (or optimizing the red:blue ratio, a species-dependent problem) also offers large benefits to the grower. However, all the treatment recommendations noted here come with nonphotosynthetic risks. Growers must consider developmental and photomorphogenic consequences of varying spectral quality that have been covered elsewhere in this article ( Fig. 4 ).
There are wider impacts of these choices to be borne in mind. For example, in the United Kingdom, recent events such as Brexit, the COVID-19 pandemic, and global logistical issues with shipping have demonstrated the fragility of supply chains across borders ( Coleman et al. 2022 ; Zurayk et al. 2022 ). LED lighting, as mentioned throughout this review, can extend the growing period of crops in greenhouses and allow for year-round production in controlled environments, increasing the production of locally grown produce, which is generally less susceptible to these issues, although not totally problem free ( Bayir et al. 2022 ).
LED lighting therefore is not only beneficial to improving crop yield and quality, but additionally supports locally grown produce and thus improves food security, while reducing carbon emissions from transportation, a metric many producers, retailers, and consumers take into account in their buying habits.
Many manufacturers focus on delivering a lighting spectrum based on a basic white or simple red and blue spectrum, while other spectral regions that are important for primary and secondary metabolism, such as green and FR, are often neglected. Here, we have laid out the foundations on the range of available lighting spectra, from the addition of FR to the balance between red and blue light, with the effects these lights have on plant growth and performance. Finally, we have also briefly investigated some of the economic benefits due to productivity increases based on the information available in the literature. Here, we have shown that the addition of FR light has the highest impact on productivity and the lowest ongoing electricity cost. The replacement of lighting such as HPS with LEDs may appear expensive compared with the predicted yield increase but there are also significant cost savings associated with the removal of HPS lights. Hardware costs for lighting continue to fall, and we believe tremendous scope remains to improve productivity, increase quality, and reduce waste in glasshouses and vertical farms through the use of tuned lighting “recipes.” As yet, no systematic survey exists in the public domain that takes advantage of the inherent dynamism offered by LED lighting to enhance yield. Thus growers and technologists are in uncharted waters where important intellectual property remains on the table that could significantly improve profitability and sustainability.
Adams, S.R. & Langton, F.A. 2005 Photoperiod and plant growth: A review J. Hortic. Sci. Biotechnol. 80 1 2 10 https://doi.org/10.1080/14620316.2005.11511882
- Search Google Scholar
- Export Citation
Affandi, F.Y. , Verdonk, J.C. , Ouzounis, T. , Ji, Y. , Woltering, E.J. & Schouten, R.E. 2020 Far-red light during cultivation induces postharvest cold tolerance in tomato fruit Postharvest Biol. Technol. 159 111019 https://doi.org/10.1016/j.postharvbio.2019.111019
Ahmed, H.A. , Yu-xin, T. & Qi-chang, Y. 2020 Lettuce plant growth and tipburn occurrence as affected by airflow using a multi-fan system in a plant factory with artificial light J. Therm. Biol. 88 102496 https://doi.org/10.1016/j.jtherbio.2019.102496
Anjana, S.U. & Iqbal, M. 2003 Nitrate accumulation in plants, factors affecting the process, and human health implications. A review Agron. Sustain. Dev. 27 45 47 https://doi.org/10.1051/agro:2006021
Bakker, J.C. , De Zwart, H.F. & Campen, J.B. 2006 Greenhouse cooling and heat recovery using fine wire heat exchangers in a closed pot plant greenhouse: Design of an energy producing greenhouse Acta Hortic. 719 263 270 https://doi.org/10.17660/ActaHortic.2006.719.29
Bayir, B. , Charles, A. , Sekhari, A. & Ouzrout, Y. 2022 Issues and challenges in short food supply chains: A systematic literature review Sustainability 14 5 3029 https://doi.org/10.3390/su14053029
Berry, J. & Bjorkman, O. 1980 photosynthetic response and adaptation to temperature in higher plants Annu. Rev. Plant Physiol. 31 1 491 543 https://doi.org/10.1146/annurev.pp.31.060180.002423
Bian, Z. , Cheng, R. , Wang, Y. , Yang, Q. & Lu, C. 2018 Effect of green light on nitrate reduction and edible quality of hydroponically grown lettuce ( Lactuca sativa L.) under short-term continuous light from red and blue light-emitting diodes Environ. Exp. Bot. 153 63 71 https://doi.org/10.1016/j.envexpbot.2018.05.010
Bian, Z. , Zhang, X. , Wang, Y. & Lu, C. 2019 Improving drought tolerance by altering the photosynthetic rate and stomatal aperture via green light in tomato ( Solanum lycopersicum L.) seedlings under drought conditions Environ. Exp. Bot. 167 103844 https://doi.org/10.1016/j.envexpbot.2019.103844
Birlanga, V. , Acosta-Motos, J.R. & Pérez-Pérez, J.M. 2021 Genotype-dependent tipburn severity during lettuce hydroponic culture is associated with altered nutrient leaf content Agronomy 11 4 616 https://doi.org/10.3390/agronomy11040616
Bliznikas, Z. , Žukauskas, A. , Samuoliene, G. , Viršile, A. , Brazaityte, A. , Jankauskiene, J. , Duchovskis, P. & Novičkovas, A. 2012 Effect of supplementary pre-harvest LED lighting on the antioxidant and nutritional properties of green vegetables Acta Hortic. 939 85 91 https://doi.org/10.17660/ActaHortic.2012.939.10
Borthwick, H.A. , Hendricks, S.B. , Parker, M.W. , Toole, E.H. & Toole, V.K. 1952 A reversible photoreaction controlling seed germination Proc. Natl. Acad. Sci. USA 38 8 662 666 https://doi.org/10.1073/pnas.38.8.662
Brazaitytė, A. , Miliauskienė, J. , Vaštakaitė-Kairienė, V. , Sutulienė, R. , Laužikė, K. , Duchovskis, P. & Małek, S. 2021 Effect of different ratios of blue and red led light on brassicaceae microgreens under a controlled environment Plants 10 4 801 https://doi.org/10.3390/plants10040801
Brown, C.S. , Schuerger, A.C. & Sager, J.C. 1995 Growth and photomorphogenesis of pepper plants under red light-emitting diodes with supplemental blue or far-red lighting J. Amer. Soc. Hort. Sci. 120 5 808 813 https://doi.org/10.21273/JASHS.120.5.808
Cao, K. , Cui, L. , Ye, L. , Zhou, X. , Bao, E. , Zhao, H. & Zou, Z. 2016 Effects of red light night break treatment on growth and flowering of tomato plants Front. Plant Sci. 7 1 8 https://doi.org/10.3389/fpls.2016.00527
Casal, J.J. 2012 Shade avoidance Arabidopsis Book 2012 10 https://doi.org/10.1199/tab.0157
Casal, J.J. 2013 Photoreceptor signaling networks in plant responses to shade Annu. Rev. Plant Biol. 64 403 427 https://doi.org/10.1146/annurev-arplant-050312-120221
Casal, J.J. , Candia, A.N. & Sellaro, R. 2014 Light perception and signalling by phytochrome A J. Expt. Bot. 65 11 2835 2845 https://doi.org/10.1093/jxb/ert379
Chalker-Scott, L. 1999 Environmental significance of anthocyanins in plant stress responses Photochem. Photobiol. 70 1 1 9 https://doi.org/10.1111/j.1751-1097.1999.tb01944.x
Chavan, S.G. , Maier, C. , Alagoz, Y. , Filipe, J.C. , Warren, C.R. , Lin, H. , Jia, B. , Loik, M.E. , Cazzonelli, C.I. , Chen, Z.H. , Ghannoum, O. & Tissue, D.T. 2020 Light-limited photosynthesis under energy-saving film decreases eggplant yield Food Energy Secur. 9 4 1 18 https://doi.org/10.1002/fes3.245
Chaves, M.M. , Pereira, J.S. , Maroco, J. , Rodrigues, M.L. , Ricardo, C.P.P. , Osório, M.L. , Carvalho, I. , Faria, T. & Pinheiro, C. 2002 How plants cope with water stress in the field. Photosynthesis and growth Ann. Bot. (Lond.) 89 7 907 916 https://doi.org/10.1093/aob/mcf105
Chia, P. & Kubota, C. 2010 End-of-day far-red light quality and dose requirements for tomato rootstock hypocotyl elongation HortScience 45 10 1501 1506 https://doi.org/10.21273/HORTSCI.45.10.1501
Choi, H.G. , Moon, B.Y. & Kang, N.J. 2015 Effects of LED light on the production of strawberry during cultivation in a plastic greenhouse and in a growth chamber Scientia Hort. 189 22 31 https://doi.org/10.1016/j.scienta.2015.03.022
Choi, K.Y. , Paek, K.Y. & Lee, Y.B. 2000 Effect of air temperature on tipburn incidence of butterhead and leaf lettuce in a plant factory 166 171 Kubota, C. & Chun, C. Transplant production in the 21st century. Springer Dordrecht, the Netherlands https://doi.org/10.1007/978-94-015-9371-7_27
Chun, C. , Toinaga, M. & Kozai, T. 2001 Floral development and bolting of spinach as affected by photoperiod and integrated photosynthetic photon flux during transplant production HortScience 36 5 889 892 https://doi.org/10.21273/hortsci.36.5.889
Coleman, P.C. , Dhaif, F. & Oyebode, O. 2022 Food shortages, stockpiling and panic buying ahead of Brexit as reported by the British media: A mixed methods content analysis BMC Public Health 22 206 https://doi.org/10.1186/s12889-022-12548-8
Courbier, S. , Snoek, B.L. , Kajala, K. , Li, L. , Van Wees, S.C.M. & Pierik, R. 2021 Mechanisms of far-red light-mediated dampening of defense against Botrytis cinerea in tomato leaves Plant Physiol. 187 3 1250 1266 https://doi.org/10.1093/plphys/kiab354
Demers, D.A. , Dorais, M. , Wien, C.H. & Gosselin, A. 1998 Effects of supplemental light duration on greenhouse tomato ( Lycopersicon esculentum Mill.) plants and fruit yields Scientia Hort. 74 4 295 306 https://doi.org/10.1016/S0304-4238(98)00097-1
Demers, D.A. & Gosselin, A. 1999 Supplemental lighting of greenhouse vegetables: Limitations and problems related to long photoperiods Acta Hortic. 481 469 473 https://doi.org/10.17660/actahortic.1999.481.54
Demmig-Adams, B. & Adams, W.W. 1992 Photoprotection and other responses of plants to high light stress Annu. Rev. Plant Physiol. Plant Mol. Biol. 43 1 599 626 https://doi.org/10.1146/annurev.pp.43.060192.003123
Department for Business Energy & Industrial Strategy 2022 Prices of fuels purchased by non-domestic consumers in the United Kingdom excluding/including CCL (QEP 3.4.1 and 3.4.2) https://www.gov.uk/government/statistical-data-sets/gas-and-electricity-prices-in-the-non-domestic-sector [accessed 8 Aug 2022]
Elkins, C. & van Iersel, M.W. 2020 Longer photoperiods with the same daily light integral improve growth of rudbeckia seedlings in a greenhouse HortScience. 55 10 1676 1682 https://doi.org/10.21273/HORTSCI15200-20
European Food Safety Authority 2008 Nitrate in vegetables—Scientific opinion of the panel on contaminants in the food chain EFSA J. 689 1 79 https://doi.org/10.2903/j.efsa.2008.689
Eurostat 2022 Gas prices for non-household consumers—bi-annual data (from 2007 onwards) https://ec.europa.eu/eurostat/web/products-datasets/-/nrg_pc_202 [accessed 8 Aug 2022]
Favre, N. , Bárcena, A. , Bahima, J.V. , Martínez, G. & Costa, L. 2018 Pulses of low intensity light as promising technology to delay postharvest senescence of broccoli Postharvest Biol. Technol. 142 107 114 https://doi.org/10.1016/j.postharvbio.2017.11.006
Ferrante, A. & Mariani, L. 2018 Agronomic management for enhancing plant tolerance to abiotic stresses: High and low values of temperature, light intensity, and relative humidity Horticulturae 4 3 21 https://doi.org/10.3390/horticulturae4030021
Firfiris, V. , Kougias, P.G. , Nikita-Martzopoulou, C. & Martzopoulos, G.G. 2012 Performance of a covered closed loop shallow geothermal greenhouse heating system Acta Hortic. 952 457 462 https://doi.org/10.17660/ActaHortic.2012.952.57
Folta, K.M. & Maruhnich, S.A. 2007 Green light: A signal to slow down or stop J. Expt. Bot. 58 12 3099 3111 https://doi.org/10.1093/jxb/erm130
Frantz, J.M. , Joly, R.J. & Mitchell, C.A. 2000 Intracanopy lighting influences radiation capture, productivity, and leaf senescence in cowpea canopies J. Amer. Soc. Hort. Sci. 125 6 694 701 https://doi.org/10.21273/jashs.125.6.694
Frantz, J.M. , Ritchie, G. , Cometti, N.N. , Robinson, J. & Bugbee, B. 2004 Exploring the limits of crop productivity: Beyond the limits of tipburn in lettuce J. Amer. Soc. Hort. Sci. 129 3 331 338 https://doi.org/10.21273/jashs.129.3.0331
Frechilla, S. , Talbott, L.D. , Bogomolni, R.A. & Zeiger, E. 2000 Reversal of blue light-stimulated stomatal opening by green light Plant Cell Physiol. 41 2 171 176 https://doi.org/10.1093/pcp/41.2.171
Garrett Owen, W. , Meng, Q. & Lopez, R.G. 2018 Promotion of flowering from far-red radiation depends on the photosynthetic daily light integral HortScience 53 4 465 471 https://doi.org/10.21273/HORTSCI12544-17
Gaudreau, L. , Charbonneau, J. , Vezina, L.P. & Gosselin, A. 1994 Photoperiod and photosynthetic photon flux influence growth and quality of greenhouse-grown lettuce HortScience 29 11 1285 1289 https://doi.org/10.21273/hortsci.29.11.1285
Gómez, C. & Mitchell, C.A. 2016a Physiological and productivity responses of high-wire tomato as affected by supplemental light source and distribution within the canopy J. Amer. Soc. Hort. Sci. 141 2 196 208 https://doi.org/10.21273/jashs.141.2.196
Gómez, C. & Mitchell, C.A. 2016b In search of an optimized supplemental lighting spectrum for greenhouse tomato production with intracanopy lighting Acta Hortic. 1134 57 62 https://doi.org/10.17660/ActaHortic.2016.1134.8
Gómez, C. , Morrow, R.C. , Bourget, C.M. , Massa, G.D. & Mitchell, C.A. 2013 Comparison of intracanopy light-emitting diode towers and overhead high-pressure sodium lamps for supplemental lighting of greenhouse-grown tomatoes HortTechnology 23 1 93 98 https://doi.org/10.21273/horttech.23.1.93
Goto, E. & Takakura, T. 1992 Supplying air to inner leaves Am Soc Agric. Engineers 35 2 641 645
Graamans, L. , Baeza, E. , van den Dobbelsteen, A. , Tsafaras, I. & Stanghellini, C. 2018 Plant factories versus greenhouses: Comparison of resource use efficiency Agric. Syst. 160 31 43 https://doi.org/10.1016/j.agsy.2017.11.003
Green-Tracewicz, E. , Page, E.R. & Swanton, C.J. 2011 Shade avoidance in soybean reduces branching and increases plant-to-plant variability in biomass and yield per plant Weed Sci. 59 1 43 49 https://doi.org/10.1614/ws-d-0-00081.1
Hamamoto, H. , Shimaji, H. & Higashide, T. 2004 Earlier-bolting spinach cultivars respond to a wider spectrum of night-break light for bolting than later-bolting cultivars Nogyo Kisho 60 3 191 195 https://doi.org/10.2480/agrmet.60.191
Hasperué, J.H. , Rodoni, L.M. , Guardianelli, L.M. , Chaves, A.R. & Martínez, G.A. 2016 Use of LED light for Brussels sprouts postharvest conservation Scientia Hortic. 213 14 281 286
Heldt, H.-W. 2005 Plant biochemistry 3rd ed. Elsevier Academic Press
Hendricks, S.B. & Borthwick, H.A. 1967 The function of phytochrome in regulation of plant growth Proc. Natl. Acad. Sci. USA 58 5 2125 2130 https://doi.org/10.1073/pnas.58.5.2125
Heo, J. , Lee, C. , Chakrabarty, D. & Paek, K. 2002 Growth responses of marigold and salvia bedding plants as affected by monochromic or mixture radiation provided by a light-emitting diode (LED) Plant Growth Regulat. 38 3 225 230 https://doi.org/10.1023/A:1021523832488
Heraut-Bron, V. , Robin, C. , Varlet-Grancher, C. , Afif, D. & Gucker, A. 1999 Light quality (red:far-red ratio): Does it affect photosynthetic activity, net CO 2 assimilation, and morphology of young white clover leaves? Can. J. Bot. 77 10 1425 1431 https://doi.org/10.1139/cjb-77-10-1425
Hernández, R. & Kubota, C. 2016 Physiological responses of cucumber seedlings under different blue and red photon flux ratios using LEDs Environ. Exp. Bot. 121 66 74 https://doi.org/10.1016/j.envexpbot.2015.04.001
Hidaka, K. , Okamoto, A. , Araki, T. , Miyoshi, Y. , Dan, K. , Imamura, H. , Kitano, M. , Sameshima, K. & Okimura, M. 2014 Effect of photoperiod of supplemental lighting with light-emitting diodes on growth and yield of strawberry Environ. Control Biol. 52 2 63 71 https://doi.org/10.2525/ecb.52.63
Higuchi, Y. , Sumitomo, K. , Oda, A. , Shimizu, H. & Hisamatsu, T. 2012 Day light quality affects the night-break response in the short-day plant chrysanthemum, suggesting differential phytochrome-mediated regulation of flowering J. Plant Physiol. 169 18 1789 1796 https://doi.org/10.1016/j.jplph.2012.07.003
Hirai, T. , Amaki, W. & Watanabe, H. 2006 Action of blue or red monochromatic light on stem internodal growth depends on plant species Acta Hortic. 711 345 349 https://doi.org/10.17660/actahortic.2006.711.47
Hoffmann, A.M. , Noga, G. & Hunsche, M. 2015 High blue light improves acclimation and photosynthetic recovery of pepper plants exposed to UV stress Environ. Exp. Bot. 109 254 263 https://doi.org/10.1016/j.envexpbot.2014.06.017
Hogewoning, S.W. , Trouwborst, G. , Maljaars, H. , Poorter, H. , van Ieperen, W. & Harbinson, J. 2010 Blue light dose—Responses of leaf photosynthesis, morphology, and chemical composition of Cucumis sativus grown under different combinations of red and blue light J. Expt. Bot. 61 11 3107 3117 https://doi.org/10.1093/jxb/erq132
Huché-thélier, L. , Crespel, L. , Le, J. , Morel, P. , Sakr, S. & Leduc, N. 2015 Light signaling and plant responses to blue and UV radiations—Perspectives for applications in horticulture Environ. Exp. Bot. 121 22 38 https://doi.org/10.1016/j.envexpbot.2015.06.009
Ilias, I.F. & Rajapakse, N. 2005 The effects of end-of-the-day red and far-red light on growth and flowering of Petunia × hybrida ‘Countdown Burgundy’ grown under photoselective films HortScience 40 1 131 133 https://doi.org/10.21273/HORTSCI.40.1.131
Islam, M.A. , Tarkowská, D. , Clarke, J.L. , Blystad, D.R. , Gislerød, H.R. , Torre, S. & Olsen, J.E. 2014 Impact of end-of-day red and far-red light on plant morphology and hormone physiology of poinsettia Scientia Hort. 174 1 77 86 https://doi.org/10.1016/j.scienta.2014.05.013
Izzo, L.G. , Mickens, M.A. , Aronne, G. & Gómez, C. 2021 Spectral effects of blue and red light on growth, anatomy, and physiology of lettuce Physiol. Plant. 172 4 2191 2202 https://doi.org/10.1111/ppl.13395
Jenkins, G.I. 2017 Photomorphogenic responses to ultraviolet-B light Plant Cell Environ. 40 11 2544 2557 https://doi.org/10.1111/pce.12934
Ji, Y. , Ouzounis, T. , Courbier, S. , Kaiser, E. , Nguyen, P.T. , Schouten, H.J. , Visser, R.G.F. , Pierik, R. , Marcelis, L.F.M. & Heuvelink, E. 2019 Far-red radiation increases dry mass partitioning to fruits but reduces Botrytis cinerea resistance in tomato Environ. Exp. Bot. 168 103889 https://doi.org/10.1016/j.envexpbot.2019.103889
Jin, W. , Urbina, J.L. , Heuvelink, E. & Marcelis, L.F.M. 2021 Adding far-red to red-blue light-emitting diode light promotes yield of lettuce at different planting densities Front. Plant Sci. 11 60997 https://doi.org/10.3389/fpls.2020.609977
Johansson, M. & Staiger, D. 2015 Time to flower: Interplay between photoperiod and the circadian clock J. Expt. Bot. 66 3 719 730 https://doi.org/10.1093/jxb/eru441
Johkan, M. , Shoji, K. , Goto, F. , Hashida, S.-N. & Yoshihara, T. 2010 Blue light-emitting diode light irradiation of seedlings improves seedling quality and growth after transplanting in red leaf lettuce HortScience 45 12 1809 1814 https://doi.org/10.21273/HORTSCI.45.12.1809
Jokinen, K. , Särkkä, L.E. & Näkkilä, J. 2012 Improving sweet pepper productivity by LED interlighting Acta Hortic 956 59 66 https://doi.org/10.17660/ActaHortic.2012.956.4
Jones-Baumgardt, C. , Llewellyn, D. & Zheng, Y. 2020 Different microgreen genotypes have unique growth and yield responses to intensity of supplemental PAR from light-emitting diodes during winter greenhouse production in southern Ontario, Canada HortScience 55 2 156 163 https://doi.org/10.21273/HORTSCI14478-19
Kaiser, E. , Matsubara, S. , Harbinson, J. , Heuvelink, E. & Marcelis, L.F.M. 2018 Acclimation of photosynthesis to lightflecks in tomato leaves: Interaction with progressive shading in a growing canopy Physiol. Plant. 162 506 517 https://doi.org/10.1111/ppl.12668
Kaiser, E. , Ouzounis, T. , Giday, H. , Schipper, R. , Heuvelink, E. & Marcelis, L.F.M. 2019a Adding blue to red supplemental light increases biomass and yield of greenhouse-grown tomatoes, but only to an optimum Front. Plant Sci. 9 2002 https://doi.org/10.3389/fpls.2018.02002
Kaiser, E. , Weerheim, K. , Schipper, R. & Dieleman, J.A. 2019b Partial replacement of red and blue by green light increases biomass and yield in tomato Scientia Hort. 249 271 279 https://doi.org/10.1016/j.scienta.2019.02.005
Kalaitzoglou, P. , van Ieperen, W. , Harbinson, J. , van der Meer, M. , Martinakos, S. , Weerheim, K. , Nicole, C.C.S. & Marcelis, L.F.M. 2019 Effects of continuous or end-of-day far-red light on tomato plant growth, morphology, light absorption, and fruit production Front. Plant Sci. 10 1 11 https://doi.org/10.3389/fpls.2019.00322
Kang, C. , Zhang, Y. , Cheng, R. , Kaiser, E. , Yang, Q. & Li, T. 2021 Acclimating cucumber plants to blue supplemental light promotes growth in full sunlight Front. Plant Sci. 12 1 14 https://doi.org/10.3389/fpls.2021.78246
Katzin, D. , Marcelis, L.F.M. & van Mourik, S. 2021 Energy savings in greenhouses by transition from high-pressure sodium to LED lighting Appl. Energy 281 116019 https://doi.org/10.1016/j.apenergy.2020.116019
Kaukoranta, T. , Särkkä, L.E. & Jokinen, K. 2017 Energy efficiency of greenhouse cucumber production under LED and HPS lighting Acta Hortic 1170 967 972 https://doi.org/10.17660/ActaHortic.2017.1170.124
Keller, M.M. , Jaillais, Y. , Pedmale, U.V. , Moreno, J.E. , Chory, J. & Ballaré, C.L. 2011 Cryptochrome 1 and phytochrome B control shade-avoidance responses in Arabidopsis via partially independent hormonal cascades Plant J. 67 2 195 207 https://doi.org/10.1111/j.1365-313X.2011.04598.x
Kim, H.H. , Goins, G.D. , Wheeler, R.M. & Sager, J.C. 2004 Green-light supplementation for enhanced lettuce growth under red-and blue-light-emitting diodes HortScience 39 7 1617 1622 https://doi.org/10.21273/hortsci.39.7.1617
Kim, H.J. , Lin, M.Y. & Mitchell, C.A. 2019a Light spectral and thermal properties govern biomass allocation in tomato through morphological and physiological changes Environ. Exp. Bot. 157 228 240 https://doi.org/10.1016/j.envexpbot.2018.10.019
Kim, H.J. , Yang, T. , Choi, S. , Wang, Y.J. , Lin, M.Y. & Liceaga, A.M. 2019b Supplemental intracanopy far-red radiation to red LED light improves fruit quality attributes of greenhouse tomatoes Scientia Hort. 261 108985 https://doi.org/10.1016/j.scienta.2019.108985
Kong, Y. , Llewellyn, D. & Zheng, Y. 2019 Response of growth, yield, and quality of edible-podded snow peas to supplemental led lighting during winter greenhouse production Can. J. Plant Sci. 99 5 676 687 https://doi.org/10.1139/cjps-2018-0288
Kovinich, N. , Kayanja, G. , Chanoca, A. , Otegui, M.S. & Grotewold, E. 2015 Abiotic stresses induce different localizations of anthocyanins in Arabidopsis Plant Signal. Behav. 10 7 2 5 https://doi.org/10.1080/15592324.2015.1027850
Kozai, T. , Niu, G. & Takagaki, M. 2019 Plant factory: An indoor vertical farming system for efficient quality food production Academic Press
Kromdijk, J. , Glowacka, K. , Leonelli, L. , Gabilly, S.T. , Iwai, M. , Niyogi, K.K. & Long, S.P. 2016 Improving photosynthesis and crop productivity by accelerating recovery Science 354 6314 857 861 https://doi.org/10.1126/science.aai8878
Kusuma, P. , Pattison, P.M. & Bugbee, B. 2020 From physics to fixtures to food: Current and potential LED efficacy Hort. Res. 7 56 https://doi.org/10.1038/s41438-020-0283-7
Kusuma, P. , Westmoreland, F.M. , Zhen, S. & Bugbee, B. 2021 Photons from NIR LEDs can delay flowering in short-day soybean and Cannabis : Implications for phytochrome activity PLoS One 16 1 17 https://doi.org/10.1371/journal.pone.0255232
Lanoue, J. , Little, C. & Hao, X. 2022 The power of far-red light at night: Photomorphogenic, physiological, and yield response in pepper during dynamic 24 hour lighting Front. Plant Sci. 13 1 19 https://doi.org/10.3389/fpls.2022.857616
Lanoue, J. , Zheng, J. , Little, C. , Grodzinski, B. & Hao, X. 2021 Continuous light does not compromise growth and yield in mini-cucumber greenhouse production with supplemental led light Plants 10 2 1 18 https://doi.org/10.3390/plants1002037
Lanoue, J. , Zheng, J. , Little, C. , Thibodea, A. , Grodzinski, B. & Hao, X. 2019 Alternating red and blue light-emitting diodes allows for injury-free tomato production with continuous lighting Front. Plant Sci. 10 1 14 https://doi.org/10.3389/fpls.2019.01114
Larsen, D.H. , Woltering, E.J. , Nicole, C.C.S. & Marcelis, L.F.M. 2020 Response of basil growth and morphology to light intensity and spectrum in a vertical farm Front. Plant Sci. 11 1 16 https://doi.org/10.3389/fpls.2020.597906
Latowski, D. , Kuczyńska, P. & Strzałka, K. 2011 Xanthophyll cycle—A mechanism protecting plants against oxidative stress Redox Report: Communications in Free Radical Research. 16 2 78 90 https://doi.org/10.1179/174329211X13020951739938
Lefsrud, M.G. , Kopsell, D.A. , Augé, R.M. & Both, A.J. 2006 Biomass production and pigment accumulation in kale grown under increasing photoperiods HortScience 41 3 603 606 https://doi.org/10.21273/hortsci.41.3.603
Legris, M. , Ince, Y.Ç. & Fankhauser, C. 2019 Molecular mechanisms underlying phytochrome-controlled morphogenesis in plants Nat. Commun. 10 5219 https://doi.org/10.1038/s41467-019-13045-0
Leivar, P. & Quail, P.H. 2011 PIFs: Pivotal components in a cellular signaling hub Trends Plant Sci. 16 1 19 28 https://doi.org/10.1016/j.tplants.2010.08.003
Liang, Y. , Kang, C. , Kaiser, E. , Kuang, Y. , Yang, Q. & Li, T. 2021 Red/blue light ratios induce morphology and physiology alterations differently in cucumber and tomato Scientia Hort. 281 109995 https://doi.org/10.1016/j.scienta.2021.109995
Lichtenthaler, H.K. & Buschmann, C. 2001 Chlorophylls and carotenoids: Measurement and characterization by UV-VIS Curr. Prot. Food Anal. Chem. 1 F4.3.1 F4.3.8 https://doi.org/10.1002/0471142913
Lichtenthaler, H.K. , Buschmann, C. & Rahmsdorf, U. 1980 The importance of blue light for the development of sun-type chloroplasts 485 494 Senger, H. The blue light syndrome. Springer Berlin and Heidelberg, Germany
Liu, J. & van Iersel, M.W. 2021 Photosynthetic physiology of blue, green, and red light: Light intensity effects and underlying mechanisms Front. Plant Sci. 12 619987 https://doi.org/10.3389/fpls.2021.619987
Lu, T. , Meng, Z. , Zhang, G. , Qi, M. , Sun, Z. , Liu, Y. & Li, T. 2017 Sub-high temperature and high light intensity induced irreversible inhibition on photosynthesis system of tomato plant ( Solanum lycopersicum L.) Front. Plant Sci. 8 1 16 https://doi.org/10.3389/fpls.2017.00365
Ma, Z. , Li, S. , Zhang, M. , Jiang, S. & Xiao, Y. 2010 Light intensity affects growth, photosynthetic capability, and total flavonoid accumulation of Anoectochilus plants HortScience 45 6 863 867 https://doi.org/10.21273/hortsci.45.6.863
Matsuda, R. , Ohashi-Kaneko, K. , Fujiwara, K. & Kurata, K. 2007 Analysis of the relationship between blue-light photon flux density and the photosynthetic properties of spinach ( Spinacia oleracea L.) leaves with regard to the acclimation of photosynthesis to growth irradiance Soil Sci. Plant Nutr. 53 4 459 465 https://doi.org/10.1111/j.1747-0765.2007.00150.x
Matthews, J.S.A. & Lawson, T. 2019 Climate change and stomatal physiology Roberts, J.A. Annual Plant Reviews online. https://doi.org/10.1002/9781119312994.apr0667
Matthews, J.S.A. , Vialet-Chabrand, S. & Lawson, T. 2020 Role of blue and red light in stomatal dynamic behaviour J. Expt. Bot. 71 7 2253 2269 https://doi.org/10.1093/jxb/erz563
Maynard, D.N. , Barker, A.V. , Minotti, P.L. & Peck, N.H. 1976 Nitrate accumulation in vegetables Adv. Agron. 28 71 118
McCree, K.J. 1971 The action spectrum, absorptance and quantum yield of photosynthesis in crop plants Agric. Meteorol. 9 191 216 https://doi.org/10.1016/0002-1571(71)90022-7
McMahon, M.J. , Kelly, J.W. , Decoteau, D.R. , Young, R.E. & Pollock, R.K. 2019 Growth of Dendranthema × grandiflorum (Ramat.) Kitamura under various spectral filters J. Am. Soc. Hort. Sci. 116 6 950 954 https://doi.org/10.21273/JASHS.116.6.950
McMaster, G.S. & Wilhelm, W.W. 1997 Growing degree-days: One equation, two interpretations Agr. For. Meteorol. 87 1 291 300
Meng, Q. , Kelly, N. & Runkle, E.S. 2019 Substituting green or far-red radiation for blue radiation induces shade avoidance and promotes growth in lettuce and kale Environ. Exp. Bot. 162 383 391 https://doi.org/10.1016/j.envexpbot.2019.03.016
Meng, Q. & Runkle, E.S. 2019 Far-red radiation interacts with relative and absolute blue and red photon flux densities to regulate growth, morphology, and pigmentation of lettuce and basil seedlings Scientia Hort. 255 269 280 https://doi.org/10.1016/j.scienta.2019.05.030
Mirkovic, T. , Ostroumov, E.E. , Anna, J.M. , Van Grondelle, R. & Govindjee Scholes, G.D. 2017 Light absorption and energy transfer in the antenna complexes of photosynthetic organisms Chem. Rev. 117 2 249 293 https://doi.org/10.1021/acs.chemrev.6b00002
Mullineaux, P.M. , Exposito-Rodriguez, M. , Laissue, P.P. & Smirnoff, N. 2018 ROS-dependent signalling pathways in plants and algae exposed to high light: Comparisons with other eukaryotes Free Radic. Biol. Med. 122 52 64 https://doi.org/10.1016/j.freeradbiomed.2018.01.033
Murage, E.N. & Masuda, M. 1997 Response of pepper and eggplant to continuous light in relation to leaf chlorosis and activities of antioxidative enzymes Scientia Hort. 70 4 269 279 https://doi.org/10.1016/S0304-4238(97)00078-2
Murakami, K. , Matsuda, R. & Fujiwara, K. 2013 Effects of supplemental lighting to a lower leaf using light-emitting diodes with different spectra on the leaf photosynthetic rate in sweet pepper Nogyo Kisho 69 2 55 63 https://doi.org/10.2480/agrmet.69.2.2
Murchie, E.H. & Lawson, T. 2013 Chlorophyll fluorescence analysis: A guide to good practice and understanding some new applications J. Expt. Bot. 64 13 3983 3998 https://doi.org/10.1093/jxb/ert208
Murchie, E.H. & Harbinson, J. 2014 Non-photochemical fluorescence quenching across scales: From chloroplasts to plants to communities 553 582 Demmig-Adams, B. , Garab, G. , Adams, W. III & Govindjee Non-photochemical quenching and energy dissipation in plants, algae and cyanobacteria. Springer Dordrecht, the Netherlands https://doi.org/10.1007/978-94-017-9032-1
Murchie, E.H. & Ruban, A.V. 2020 Dynamic non-photochemical quenching in plants: From molecular mechanism to productivity Plant J. 101 4 885 896 https://doi.org/10.1111/tpj.14601
Nagendran, R. & Lee, Y.H. 2015 Green and red light reduces the disease severity by Pseudomonas cichorii JBC1 in tomato plants via upregulation of defense-related gene expression Phytopathology 105 4 412 418 https://doi.org/10.1094/PHYTO-04-14-0108-R
Nascimento, L.B.D.S. , Brunetti, C. , Agati, G. , Iacono, C.L. , Detti, C. , Giordani, E. , Ferrini, F. & Gori, A. 2020 Short-term pre-harvest uv-b supplement enhances the polyphenol content and antioxidant capacity of Ocimum basilicum leaves during storage Plants 9 6 1 19 https://doi.org/10.3390/plants9060797
Naznin, M.T. , Lefsrud, M. , Gravel, V. & Azad, M.O.K. 2019 Blue light added with red LEDs enhance growth characteristics, pigments content, and antioxidant capacity in lettuce, spinach, kale, basil, and sweet pepper in a controlled environment Plants 8 4 93 https://doi.org/10.3390/plants8040093
Nelson, J.A. & Bugbee, B. 2014 Economic analysis of greenhouse lighting: Light emitting diodes vs. high intensity discharge fixtures PLoS One 9 6 e99010 https://doi.org/10.1371/journal.pone.0099010
Ngcobo, B.L. , Bertling, I. & Clulow, A.D. 2020 Preharvest illumination of cherry tomato reduces ripening period, enhances fruit carotenoid concentration and overall fruit quality J. Hortic. Sci. Biotechnol. 95 5 617 627 https://doi.org/10.1080/14620316.2020.1743771
Nguyen, T.P.D. , Tran, T.T.H. & Nguyen, Q.T. 2019 Effects of light intensity on the growth, photosynthesis and leaf microstructure of hydroponic cultivated spinach ( Spinacia oleracea L.) under a combination of red and blue LEDs in house Agric. Technol. Thail. 15 1 75 90
Nicole, C.C.S. , Charalambous, F. , Martinakos, S. , Van De Voort, S. , Li, Z. , Verhoog, M. & Krijn, M. 2016 Lettuce growth and quality optimization in a plant factory Acta Hortic. 1134 231 238 https://doi.org/10.17660/ActaHortic.2016.1134.31
Ohtake, N. , Ishikura, M. , Suzuki, H. , Yamori, W. & Goto, E. 2018 Continuous irradiation with alternating red and blue light enhances plant growth while keeping nutritional quality in lettuce HortScience 53 12 1804 1809 https://doi.org/10.21273/HORTSCI13469-18
Ouzounis, T. , Heuvelink, E. , Ji, Y. , Schouten, H.J. , Visser, R.G.F. & Marcelis, L.F.M. 2016 Blue and red LED lighting effects on plant biomass, stomatal conductance, and metabolite content in nine tomato genotypes Acta Hortic. 1134 251 258 https://doi.org/10.17660/ActaHortic.2016.1134.34
Palmer, S. & van Iersel, M.W. 2020 Increasing growth of lettuce and mizuna under sole-source LED lighting using longer photoperiods with the same daily light integral Agronomy 10 11 1659 https://doi.org/10.3390/agronomy10111659
Park, Y. & Runkle, E.S. 2019 Blue radiation attenuates the effects of the red to far-red ratio on extension growth but not on flowering Environ. Exp. Bot. 168 103871 https://doi.org/10.1016/j.envexpbot.2019.103871
Pattison, P.M. , Tsao, J.Y. , Brainard, G.C. & Bugbee, B. 2018 LEDs for photons, physiology and food Nature 563 7732 493 500 https://doi.org/10.1038/s41586-018-0706-x
Pedmale, U.V. , Huang, S.S.C. , Zander, M. , Cole, B.J. , Hetzel, J. , Ljung, K. , Reis, P.A.B. , Sridevi, P. , Nito, K. , Nery, J.R. , Ecker, J.R. & Chory, J. 2016 Cryptochromes interact directly with PIFs to control plant growth in limiting blue light Cell 164 1–2 233 245 https://doi.org/10.1016/j.cell.2015.12.018
Pennisi, G. , Orsini, F. , Landolfo, M. , Pistillo, A. , Crepaldi, A. , Nicola, S. , Fernández, J.A. , Marcelis, L.F.M. & Gianquinto, G. 2020 Optimal photoperiod for indoor cultivation of leafy vegetables and herbs Eur. J. Hortic. Sci. 85 5 329 338 https://doi.org/10.17660/eJHS.2020/85.5.4
Pennisi, G. , Blasioli, S. , Cellini, A. , Maia, L. , Crepaldi, A. , Braschi, I. , Spinelli, F. , Nicol, S. , Fernandez, J.A. , Stanghellini, C. , Marcelis, L.F.M. , Orsini, F. & Gianquinto, G. 2019 Unraveling the role of red:blue LED lights on resource use efficiency and nutritional properties of indoor grown sweet basil Front. Plant Sci. 10 1 14 https://doi.org/10.3389/fpls.2019.00305
Peterman, E.J.G. , Gradinaru, C.C. , Calkoen, F. , Borst, J.C. , Van Grondelle, R. & Van Amerongen, H. 1997 Xanthophylls in light-harvesting complex II of higher plants: Light harvesting and triplet quenching Biochemistry 36 40 12208 12215 https://doi.org/10.1021/bi9711689
Pettai, H. , Oja, V. , Freiber, A. & Laisk, A. 2005 Photosynthetic activity of far-red light in green plants Biochim. Biophys. Acta Bioenerg. 1708 3 311 321 https://doi.org/10.1016/j.bbabio.2005.05.005
Pettersen, R.I. , Torre, S. & Gislerød, H.R. 2010 Effects of intracanopy lighting on photosynthetic characteristics in cucumber Scientia Hort. 125 2 77 81 https://doi.org/10.1016/j.scienta.2010.02.006
Piovene, C. , Orsini, F. , Bosi, S. , Sanoubar, R. , Bregola, V. , Dinelli, G. & Gianquinto, G. 2015 Optimal Red: Blue ratio in led lighting for nutraceutical indoor horticulture Scientia Hort. 193 202 208 https://doi.org/10.1016/j.scienta.2015.07.015
Proietti, S. , Moscatello, S. , Riccio, F. , Downey, P. & Battistelli, A. 2021 continuous lighting promotes plant growth, light conversion efficiency, and nutritional quality of Eruca vesicaria (L.) Cav. in controlled environment with minor effects due to light quality Front. Plant Sci. 12 https://doi.org/10.3389/fpls.2021.730119
Qian, M. , Rosenqvist, E. , Flygate, A.-M. , Kalbina, I. , Teng, Y. , Jansen, M.A.K. & Strid, Å. 2020 UV-A light induces a robust and dwarfed phenotype in cucumber plants ( Cucumis sativus L.) without affecting fruit yield Scientia Hort. 263 109110 https://doi.org/10.1016/j.scienta.2019.109110
Rabara, R.C. , Behrman, G. , Timbol, T. & Rushton, P.J. 2017 Effect of spectral quality of monochromatic LED lights on the growth of artichoke seedlings Front. Plant Sci. 8 1 9 https://doi.org/10.3389/fpls.2017.00190
Rahman, M.Z. , Honda, Y. & Arase, S. 2003 Red-light-induced resistance in broad bean ( Vicia faba L.) to Leaf spot disease caused by Alternaria tenuissima J. Phytopathol. 151 2 86 91 https://doi.org/10.1046/j.1439-0434.2003.00685.x
Sago, Y. 2016 Effects of light intensity and growth rate on tipburn development and leaf calcium concentration in butterhead lettuce HortScience 51 9 1087 1091 https://doi.org/10.21273/HORTSCI10668-16
Särkkä, L.E. , Jokinen, K. , Ottosen, C.O. & Kaukoranta, T. 2017 Effects of HPS and LED lighting on cucumber leaf photosynthesis, light quality penetration and temperature in the canopy, plant morphology and yield Agric. Food Sci. 26 2 101 109 https://doi.org/10.23986/afsci.60293
Schenkels, L. , Saeys, W. , Lauwers, A. & De Proft, M.P. 2020 Green light induces shade avoidance to alter plant morphology and increases biomass production in Ocimum basilicum L Scientia Hort. 261 109002 https://doi.org/10.1016/j.scienta.2019.109002
Sellaro, R. , Crepy, M. , Trupkin, S.A. , Karayekov, E. , Buchovsky, A.S. , Rossi, C. & Casal, J.J. 2010 Cryptochrome as a sensor of the blue/green ratio of natural radiation in Arabidopsis Plant Physiol. 154 1 401 409 https://doi.org/10.1104/pp.110.160820
Shibaeva, T.G. , Sherudilo, E.G. , Rubaeva, A.A. & Titov, A.F. 2022 Continuous LED lighting enhances yield and nutritional value of four genotypes of Brassicaceae microgreens Plants 11 2 1 14 https://doi.org/10.3390/plants11020176
Shibuya, T. , Itagaki, K. , Tojo, M. , Endo, R. & Kitaya, Y. 2011 Fluorescent illumination with high red-to-far-red ratio improves resistance of cucumber seedlings to powdery mildew HortScience 46 3 429 431 https://doi.org/10.21273/hortsci.46.3.429
Signore, A. , Bell, L. , Santamaria, P. , Wagstaff, C. & Van Labeke, M.-C. 2020 Red light is effective in reducing nitrate concentration in rocket by increasing nitrate reductase activity, and contributes to increased total glucosinolates content Front. Plant Sci. 11 604 https://doi.org/10.3389/fpls.2020.00604
Siomos, A.S. & Koukounaras, A. 2007 Quality and Postharvest Physiology of Rocket Leaves Fresh Prod. 1 1 59 65
Smith, H. & Whitelam, G.C. 1997 The shade avoidance syndrome: Multiple responses mediated by multiple phytochromes Plant Cell Environ. 20 6 840 844 https://doi.org/10.1046/j.1365-3040.1997.d01-104.x
Smith, H.L. , Mcausland, L. & Murchie, E.H. 2017 Don’t ignore the green light: Exploring diverse roles in plant processes J. Expt. Bot. 68 9 2099 2110 https://doi.org/10.1093/jxb/erx098
Snowden, M.C. , Cope, K.R. & Bugbee, B. 2016 Sensitivity of seven diverse species to blue and green light: Interactions with photon flux PLoS One 11 10 1 32 https://doi.org/10.1371/journal.pone.0163121
Son, K.H. & Oh, M.M. 2015 Growth, photosynthetic and antioxidant parameters of two lettuce cultivars as affected by red, green, and blue light-emitting diodes Hort. Environ. Biotechnol. 56 5 639 653 https://doi.org/10.1007/s13580-015-1064-3
Sørensen, H.K. , Fanourakis, D. , Tsaniklidis, G. , Bouranis, D. , Nejad, A.R. & Ottosen, C.O. 2020 Using artificial lighting based on electricity price without a negative impact on growth, visual quality or stomatal closing response in Passiflora Scientia Hort. 267 109354 https://doi.org/10.1016/j.scienta.2020.109354
Stamatakis, K. , Tsimilli-Michael, M. & Papageorgiou, G.C. 2014 On the question of the light-harvesting role of β-carotene in photosystem II and photosystem I core complexes Plant Physiol. Biochem. 81 121 127 https://doi.org/10.1016/j.plaphy.2014.01.014
Stevens, J. , Faralli, M. , Wall, S. , Stamford, J.D. & Lawson, T. 2021 Stomatal responses to climate change Becklin, K. , Ward, K. & Way, D.A. Photosynthesis, respiration and climate change. Springer https://doi.org/10.1007/978-3-030-64926-5
Suthaparan, A. , Torre, S. , Stensvand, A. , Herrero, M.L. , Pettersen, R.I. , Gadoury, D.M. & Gislerød, H.R. 2010 Specific light-emitting diodes can suppress sporulation of Podosphaera pannosa on greenhouse roses Plant Dis. 94 9 1105 1110 https://doi.org/10.1094/PDIS-94-9-1105
Tantasawat, P.A. , Sorntip, A. & Pornbungkerd, P. 2015 Effects of exogenous application of plant growth regulators on growth, yield, and in vitro gynogenesis in cucumber HortScience 50 3 372 382 https://doi.org/10.21273/hortsci.50.3.374
Terashima, I. , Fujita, T. , Inoue, T. , Chow, W.S. & Oguchi, R. 2009 Green light drives leaf photosynthesis more efficiently than red light in strong white light: Revisiting the enigmatic question of why leaves are green Plant Cell Physiol. 50 4 684 697 https://doi.org/10.1093/pcp/pcp034
Tewolde, F.T. , Shiina, K. , Maruo, T. , Takagaki, M. , Kozai, T. & Yamori, W. 2018 Supplemental LED inter-lighting compensates for a shortage of light for plant growth and yield under the lack of sunshine PLoS One 13 11 1 14 https://doi.org/10.1371/journal.pone.0206592
Thoma, F. , Somborn-Schulz, A. , Schlehuber, D. & Keuter, V. 2020 Effects of light on secondary metabolites in selected leafy greens: A review Plant Metabolism and Chemodiversity 11 1 15 https://doi.org/10.3389/fpls.2020.00497
Topcu, Y. , Dogan, A. , Sahin-Nadeem, H. , Polat, E. , Kasimoglu, Z. & Erkan, M. 2018 Morphological and biochemical responses of broccoli florets to supplemental ultraviolet-B illumination Agric. Ecosyst. Environ. 259 1 10 https://doi.org/10.1016/j.agee.2018.02.027
Touliatos, D. , Dodd, I.C. & Mcainsh, M. 2016 Vertical farming increases lettuce yield per unit area compared to conventional horizontal hydroponics Food Energy Secur. 5 3 184 191 https://doi.org/10.1002/fes3.83
Trouwborst, G. , Hogewoning, S.W. , van Kooten, O. , Harbinson, J. & van Ieperen, W. 2016 Plasticity of photosynthesis after the “red light syndrome” in cucumber Environ. Exp. Bot. 121 5 82 https://doi.org/10.1016/j.envexpbot.2015.05.002
Trouwborst, G. , Schapendonk, A.H.C.M. , Rappoldt, K. , Pot, S. , Hogewoning, S.W. & van Ieperen, W. 2011 The effect of intracanopy lighting on cucumber fruit yield—Model analysis Scientia Hort. 129 2 273 278 https://doi.org/10.1016/j.scienta.2011.03.042
Van Gestel, N.C. , Nesbit, A.D. , Gordon, E.P. , Green, C. , Paré, P.W. , Thompson, L. , Peffley, E.B. & Tissue, D.T. 2005 Continuous light may induce photosynthetic downregulation in onion—Consequences for growth and biomass partitioning Physiol. Plant. 125 2 235 246 https://doi.org/10.1111/j.1399-3054.2005.00560.x
Velez-Ramirez, A.I. , van Ieperen, W. , Vreugdenhil, D. & Millenaar, F.F. 2014 Continuous-light tolerance in tomato is graft-transferable Planta 241 1 285 290 https://doi.org/10.1007/s00425-014-2202-3
Velez-Ramirez, A.I. , van Ieperen, W. , Vreugdenhil, D. & Millenaar, F.F. 2015 Continuous-light tolerance in tomato is graft-transferable Planta 241 1 285 290 https://doi.org/10.1007/s00425-014-2202-3
Vialet-Chabrand, S. , Matthews, J.S.A. , Simkin, A.J. , Raines, C.A. & Lawson, T. 2017 Importance of fluctuations in light on plant photosynthetic acclimation Plant Physiol. 173 4 2163 2179 https://doi.org/10.1104/pp.16.01767
Walters, K.J. & Lopez, R.G. 2021 Modeling growth and development of hydroponically grown dill, parsley, and watercress in response to photosynthetic daily light integral and mean daily temperature PLoS One 16 1 16 https://doi.org/10.1371/journal.pone.0248662
Wan, Y. & Folta, K.M. 2013 Contributions of green light to plant growth and development Amer. J. Bot. 100 1 70 78 https://doi.org/10.3732/ajb.1200354
Wang, C. , He, J. , Zhao, T.H. , Cao, Y. , Wang, G. , Sun, B. , Yan, X. , Guo, W. & Li, M.H. 2019 The smaller the leaf is, the faster the leaf water loses in a temperate forest Front. Plant Sci. 10 1 12 https://doi.org/10.3389/fpls.2019.00058
Wang, F. , Guo, Z. , Li, H. , Wang, M. , Onac, E. , Zhou, J. , Xia, X. , Shi, K. , Yu, J. & Zhou, Y. 2016a Phytochrome a and b function antagonistically to regulate cold tolerance via abscisic acid-dependent jasmonate signaling Plant Physiol. 170 1 459 471 https://doi.org/10.1104/pp.15.01171
Wang, H. , Jiang, Y.P. , Yu, H.J. , Xia, X.J. , Shi, K. , Zhou, Y.H. & Yu, J.Q. 2010 Light quality affects incidence of powdery mildew, expression of defence-related genes and associated metabolism in cucumber plants Eur. J. Plant Pathol. 127 1 125 135 https://doi.org/10.1007/s10658-009-9577-1
Wang, H. , Zhang, Z. , Li, H. , Zhao, X. , Liu, X. , Ortiz, M. , Lin, C. & Liu, B. 2013 CONSTANS-LIKE 7 regulates branching and shade avoidance response in Arabidopsis J. Expt. Bot. 64 4 1017 1024 https://doi.org/10.1093/jxb/ers376
Wang, J. , Lu, W. , Tong, Y. & Yang, Q. 2016b Leaf morphology, photosynthetic performance, chlorophyll fluorescence, stomatal development of lettuce ( Lactuca sativa L.) exposed to different ratios of red light to blue light Front. Plant Sci. 7 1 10 https://doi.org/10.3389/fpls.2016.00250
Wanlai, Z. , Wenke, L. & Qichang, Y. 2013 Reducing nitrate content in lettuce by pre-harvest continuous light delivered by red and blue light-emitting diodes J. Plant Nutr. 36 3 481 490 https://doi.org/10.1080/01904167.2012.748069
Wargent, J.J. , Elfadly, E.M. , Moore, J.P. & Paul, N.D. 2011 Increased exposure to UV-B radiation during early development leads to enhanced photoprotection and improved long-term performance in Lactuca sativa Plant Cell Environ. 34 8 1401 1413 https://doi.org/10.1111/j.1365-3040.2011.02342.x
Weaver, G. & van Iersel, M.W. 2020 Longer photoperiods with adaptive lighting control can improve growth of greenhouse-grown ‘Little Gem’ lettuce ( Lactuca sativa ) HortScience 55 4 573 580 https://doi.org/10.21273/HORTSCI14721-19
Wollaeger, H.M. & Runkle, E.S. 2015 Growth and acclimation of impatiens, salvia, petunia, and tomato seedlings to blue and red light HortScience 50 4 522 529 https://doi.org/10.21273/hortsci.50.4.522
Yan, Z. , He, D. , Niu, G. , Zhou, Q. & Qu, Y. 2019 Growth, nutritional quality, and energy use efficiency of hydroponic lettuce as influenced by daily light integrals exposed to white versus white plus red light-emitting diodes HortScience 54 10 1737 1744 https://doi.org/10.21273/HORTSCI14236-19
Yang, F. , Liu, Q. , Cheng, Y. , Feng, L. , Wu, X. , Fan, Y. , Raza, M.A. , Wang, X. , Yong, T. , Liu, W. , Liu, J. , Du, J. , Shu, K. & Yang, W. 2020 Low red/far-red ratio as a signal promotes carbon assimilation of soybean seedlings by increasing the photosynthetic capacity BMC Plant Biol. 20 1 1 12 https://doi.org/10.1186/s12870-020-02352-0
Yang, S. , Logan, J. & Coffey, D.L. 1995 Mathematical formulae for calculating the base temperature for growing degree days Agr. For. Meteorol. 74 1–2 61 74 https://doi.org/10.1016/0168-1923(94)02185-M
Yang, Y.X. , Wang, M.M. , Yin, Y.L. , Onac, E. , Zhou, G.F. , Peng, S. , Xia, X.J. , Shi, K. , Yu, J.Q. & Zhou, Y.H. 2015 RNA-seq analysis reveals the role of red light in resistance against Pseudomonas syringae pv. tomato DC3000 in tomato plants BMC Genomics 16 1 1 16 https://doi.org/10.1186/s12864-015-1228-7
Yang, Y.X. , Wu, C. , Ahammed, G.J. , Wu, C. , Yang, Z. , Wan, C. & Chen, J. 2018 Red light-induced systemic resistance against root-knot nematode is mediated by a coordinated regulation of salicylic acid, jasmonic acid and redox signaling in watermelon Front. Plant Sci. 9 1 13 https://doi.org/10.3389/fpls.2018.00899
Ying, Q. , Kong, Y. , Jones-Baumgardt, C. & Zheng, Y. 2020a Responses of yield and appearance quality of four Brassicaceae microgreens to varied blue light proportion in red and blue light-emitting diodes lighting Scientia Hort. 259 108857 https://doi.org/10.1016/j.scienta.2019.108857
Ying, Q. , Kong, Y. & Zheng, Y. 2020b Overnight supplemental blue, rather than far-red, light improves microgreen yield and appearance quality without compromising nutritional quality during winter greenhouse production HortScience 55 9 1468 1474 https://doi.org/10.21273/HORTSCI15196-20
Zahedi, S.M. & Sarikhani, H. 2016 Effect of far-red light, temperature, and plant age on morphological changes and induction of flowering of a ‘June-bearing’ strawberry Hort. Environ. Biotechnol. 57 4 340 347 https://doi.org/10.1007/s13580-016-0018-8
Zhang, T. , Maruhnich, S.A. & Folta, K.M. 2011 Green light induces shade avoidance symptoms Plant Physiol. 157 3 1528 1536 https://doi.org/10.1104/pp.111.180661
Zhang, Y. , Kaiser, E. , Zhang, Y. , Zou, J. , Bian, Z. , Yang, Q. & Li, T. 2020 UVA radiation promotes tomato growth through morphological adaptation leading to increased light interception Environ. Exp. Bot. 176 104073 https://doi.org/10.1016/j.envexpbot.2020.104073
Zhang, Y. , Kaiser, E. , Zhang, Y. , Yang, Q. & Li, T. 2019 Red/blue light ratio strongly affects steady-state photosynthesis, but hardly affects photosynthetic induction in tomato ( Solanum lycopersicum ) Physiol. Plant. 167 2 144 158 https://doi.org/10.1111/ppl.12876
Zhen, S. & Bugbee, B. 2020a Far-red photons have equivalent efficiency to traditional photosynthetic photons: Implications for redefining photosynthetically active radiation Plant Cell Environ. 43 5 1259 1272 https://doi.org/10.1111/pce.13730
Zhen, S. & Bugbee, B. 2020b Substituting far-red for traditionally defined photosynthetic photons results in equal canopy quantum yield for CO 2 fixation and increased photon capture during long-term studies: Implications for re-defining PAR Front. Plant Sci. 11 1 14 https://doi.org/10.3389/fpls.2020.581156
Zhen, S. , van Iersel, M.W. & Bugbee, B. 2022 Photosynthesis in sun and shade: The surprising importance of far-red photon New Phytol. 236 538 546 https://doi.org/10.1111/nph.18375
Zhen, S. , van Iersel, M. & Bugbee, B. 2021 Why far-red photons should be included in the definition of photosynthetic photons and the measurement of horticultural fixture efficacy Front. Plant Sci. 12 10 13 https://doi.org/10.3389/fpls.2021.693445
Zhen, S. & van Iersel, M.W. 2017 Far-red light is needed for efficient photochemistry and photosynthesis J. Plant Physiol. 209 115 122 https://doi.org/10.1016/j.jplph.2016.12.004
Zheng, X.T. , Yu, Z.C. , Tang, J.W. , Cai, M.L. , Chen, Y.L. , Yang, C.W. , Chow, W.S. & Peng, C.L. 2020 The major photoprotective role of anthocyanins in leaves of Arabidopsis thaliana under long-term high light treatment: Antioxidant or light attenuator? Photosynth. Res. 148 25 40 https://doi.org/10.1007/s11120-020-00761-8
Zheng, Y. , Zhang, Y. , Liu, H. , Li, Y. , Liu, Y. , Hao, Y. & Lei, B. 2018 Supplemental blue light increases growth and quality of greenhouse pak choi depending on cultivar and supplemental light intensity J. Integr. Agric. 17 10 2245 2256 https://doi.org/10.1016/S2095-3119(18)62064-7
Zhou, J. , Li, P.P. , Wang, J.Z. & Fu, W. 2019 Growth, photosynthesis, and nutrient uptake at different light intensities and temperatures in lettuce HortScience 54 11 1925 1933 https://doi.org/10.21273/HORTSCI14161-19
Zou, T. , Huang, C. , Wu, P. , Ge, L. & Xu, Y. 2020 Optimization of artificial light for spinach growth in plant factory based on orthogonal test Plants 9 4 490 https://doi.org/10.3390/plants9040490
Zurayk, R. , Yehya, A.A.K. & Bahn, R.A. 2022 Fragility and resilience in food systems: What can we learn from the COVID-19 crisis? Shaw, R. & Gurtoo, A. Global Pandemic and Human Security. Springer Singapore https://doi.org/10.1007/978-981-16-5074-1_10
Supplementary Materials
- Supplemental Material 1 (XLSX 88.0 KB)
- Supplemental Material 2 (XLSX 248 KB)
- Supplemental Material 3 (XLSX 72.0 KB)
- Supplemental Material 4 (DOCX 80.0 KB)
Contributor Notes
This work was supported by Hy4Dense, a project that has received funding from the Interreg 2 Seas programme 2014-2020, co-funded by the European Regional Development Fund under subsidy contract no. 2S05-030. Phillip A. Davey is thanked for spectral measurements of light sources.
Current address of J.S.: Vertical Future, 3 Creekside, London, SE8 4SA, United Kingdom.
T.L. is the corresponding author. E-mail: [email protected] .
All Time | Past Year | Past 30 Days | |
---|---|---|---|
Abstract Views | 0 | 0 | 0 |
Full Text Views | 7333 | 4108 | 1016 |
PDF Downloads | 5534 | 2624 | 240 |
Headquarters:
1018 Duke Street
Alexandria, VA 22314
Phone : 703.836.4606
Email : [email protected]
© 2018-2024 American Society for Horticultural Science
- [185.80.151.9]
- 185.80.151.9
Character limit 500 /500
Thank you for visiting nature.com. You are using a browser version with limited support for CSS. To obtain the best experience, we recommend you use a more up to date browser (or turn off compatibility mode in Internet Explorer). In the meantime, to ensure continued support, we are displaying the site without styles and JavaScript.
- View all journals
- Explore content
- About the journal
- Publish with us
- Sign up for alerts
- Review Article
- Published: 23 August 2021
Emerging light-emitting diodes for next-generation data communications
- Aobo Ren ORCID: orcid.org/0000-0001-5133-2775 1 , 2 na1 ,
- Hao Wang ORCID: orcid.org/0000-0002-8976-1468 3 na1 ,
- Wei Zhang ORCID: orcid.org/0000-0002-2678-8372 2 ,
- Jiang Wu ORCID: orcid.org/0000-0003-0679-6196 1 , 4 ,
- Zhiming Wang 1 ,
- Richard V. Penty 3 &
- Ian H. White 3 , 5
Nature Electronics volume 4 , pages 559–572 ( 2021 ) Cite this article
7370 Accesses
126 Citations
61 Altmetric
Metrics details
- Lasers, LEDs and light sources
- Optics and photonics
The continuing development of consumer electronics, mobile communications and advanced computing technologies has led to a rapid growth in data traffic, creating challenges for the communications industry. Light-emitting diode (LED)-based communication links are of potential use in both free space and optical interconnect applications, and LEDs based on emerging semiconductor materials, which can offer tunable optoelectronics properties and solution-processable manufacturing, are of particular interest in the development of next-generation data communications. Here we review the development of emerging LED materials—organic semiconductors, colloidal quantum dots and metal halide perovskites—for use in optical communications. We examine efforts to improve the modulation performance and device efficiency of these LEDs, and consider potential applications in on-chip interconnects and light fidelity (Li-Fi). We also explore the challenges that exist in developing practical high-speed LED-based data communication systems.
This is a preview of subscription content, access via your institution
Access options
Access Nature and 54 other Nature Portfolio journals
Get Nature+, our best-value online-access subscription
24,99 € / 30 days
cancel any time
Subscribe to this journal
Receive 12 digital issues and online access to articles
111,21 € per year
only 9,27 € per issue
Buy this article
- Purchase on SpringerLink
- Instant access to full article PDF
Prices may be subject to local taxes which are calculated during checkout
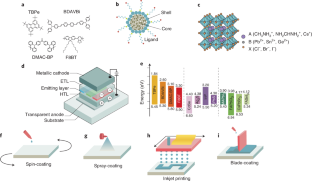
Similar content being viewed by others
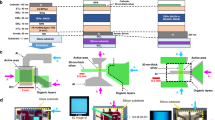
245 MHz bandwidth organic light-emitting diodes used in a gigabit optical wireless data link
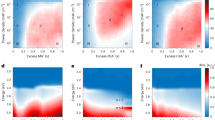
High-bandwidth perovskite photonic sources on silicon
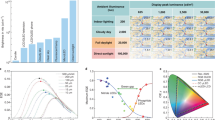
Micro light-emitting diodes
Lindemann, M. et al. Ultrafast spin-lasers. Nature 568 , 212–215 (2019).
Article Google Scholar
Karunatilaka, D., Zafar, F., Kalavally, V. & Parthiban, R. LED based indoor visible light communications: state of the art. IEEE Commun. Surv. Tutorials 17 , 1649–1678 (2015).
Rappaport, T. S. et al. Wireless communications and applications above 100 GHz: opportunities and challenges for 6G and beyond. IEEE Access 7 , 78729–78757 (2019).
Jovicic, A., Li, J. & Richardson, T. Visible light communication: opportunities, challenges and the path to market. IEEE Commun. Mag. 51 , 26–32 (2013).
Dang, S., Amin, O., Shihada, B. & Alouini, M.-S. What should 6G be? Nat. Electron. 3 , 20–29 (2020).
Ren, A., Yuan, L., Xu, H., Wu, J. & Wang, Z. Recent progress of III–V quantum dot infrared photodetectors on silicon. J. Mater. Chem. C 7 , 14441–14453 (2019).
Rashidi, A., Monavarian, M., Aragon, A., Rishinaramangalam, A. & Feezell, D. Nonpolar plane InGaN/GaN micro-scale light-emitting diode with 1.5 GHz modulation bandwidth. IEEE Electron Device Lett. 39 , 520–523 (2018).
Wang, L. et al. 13 GHz E-O bandwidth GaN-based micro-LED for multi-gigabit visible light communication. Photon. Res. 9 , 792 (2021).
Clark, J. & Lanzani, G. Organic photonics for communications. Nat. Photon. 4 , 438–446 (2010).
García De Arquer, F. P., Armin, A., Meredith, P. & Sargent, E. H. Solution-processed semiconductors for next-generation photodetectors. Nat. Rev. Mater. 2 , 1–16 (2017).
Google Scholar
Asghari, M. & Krishnamoorthy, A. V. Energy-efficient communication. Nat. Photon. 5 , 268–270 (2011).
Tang, C. W. & Vanslyke, S. A. Organic electroluminescent diodes. Appl. Phys. Lett. 51 , 913–915 (1987).
Burroughes, J. H. et al. Light-emitting diodes based on conjugated polymers. Nature 347 , 539–541 (1990).
Jou, J. H., Kumar, S., Agrawal, A., Li, T. H. & Sahoo, S. Approaches for fabricating high efficiency organic light emitting diodes. J. Mater. Chem. C 3 , 2974–3002 (2015).
Colvin, V. L., Schlamp, M. C. & Alivisatos, A. P. Light-emitting diodes made from cadmium selenide nanocrystals and a semiconducting polymer. Nature 370 , 354–357 (1994).
Dai, X. et al. Solution-processed, high-performance light-emitting diodes based on quantum dots. Nature 515 , 96–99 (2014).
Won, Y. H. et al. Highly efficient and stable InP/ZnSe/ZnS quantum dot light-emitting diodes. Nature 575 , 634–638 (2019).
Shirasaki, Y., Supran, G. J., Bawendi, M. G. & Bulović, V. Emergence of colloidal quantum-dot light-emitting technologies. Nat. Photon. 7 , 13–23 (2013).
Quan, L. N. et al. Tailoring the energy landscape in quasi-2D halide perovskites enables efficient green-light emission. Nano Lett. 17 , 3701–3709 (2017).
Kojima, A., Teshima, K., Shirai, Y. & Miyasaka, T. Organometal halide perovskites as visible-light sensitizers for photovoltaic cells. J. Am. Chem. Soc. 131 , 6050–6051 (2009).
Best research-cell efficiency chart. National Renewable Energy Laboratory https://www.nrel.gov/pv/cell-efficiency.html (2021).
Lin, K. et al. Perovskite light-emitting diodes with external quantum efficiency exceeding 20 per cent. Nature 562 , 245–248 (2018).
Chiba, T. et al. Anion-exchange red perovskite quantum dots with ammonium iodine salts for highly efficient light-emitting devices. Nat. Photon. 12 , 681–687 (2018).
Cao, Y. et al. Perovskite light-emitting diodes based on spontaneously formed submicrometre-scale structures. Nature 562 , 249–253 (2018).
Zhao, B. et al. High-efficiency perovskite–polymer bulk heterostructure light-emitting diodes. Nat. Photon. 12 , 783–789 (2018).
Kim, Y. H., Cho, H. & Lee, T. W. Metal halide perovskite light emitters. Proc. Natl. Acad. Sci. USA 113 , 11694–11702 (2016).
Barlow, I. A., Kreouzis, T. & Lidzey, D. G. A polymer light-emitting diode as an optical communication light source. Org. Electron. 8 , 621–624 (2007).
Fukuda, T., Okada, T., Wei, B., Ichikawa, M. & Taniguchi, Y. Influence of carrier-injection efficiency on modulation rate of organic light source. Opt. Lett. 32 , 1905 (2007).
Fukuda, T., Wei, B., Suto, E., Ichikawa, M. & Taniguchi, Y. Fast-response organic-inorganic hybrid light-emitting diode. Phys. Status Solidi Rapid Res. Lett. 2 , 290–292 (2008).
Barlow, I. A., Kreouzis, T. & Lidzey, D. G. High-speed electroluminescence modulation of a conjugated-polymer light emitting diode. Appl. Phys. Lett. 94 , 11–14 (2009).
Haigh, P. A. et al. Visible light communications: real time 10 Mb/s link with a low bandwidth polymer light-emitting diode. Opt. Express 22 , 2830 (2014).
Haigh, P. A. et al. Wavelength-multiplexed polymer LEDs: towards 55 Mb/s organic visible light communications. IEEE J. Sel. Areas Commun. 33 , 1819–1828 (2015).
Chen, H., Xu, Z., Gao, Q. & Li, S. A 51.6 Mb/s experimental VLC system using a monochromic organic LED. IEEE Photon. J. 10 , 1–12 (2018).
Minotto, A. et al. Visible light communication with efficient far-red/near-infrared polymer light-emitting diodes. Light Sci. Appl. 9 , 70 (2020).
Liu, Y., Li, C., Ren, Z., Yan, S. & Bryce, M. R. All-organic thermally activated delayed fluorescence materials for organic light-emitting diodes. Nat. Rev. Mater. 3 , 18020 (2018).
Xu, Z., Tang, B. Z., Wang, Y. & Ma, D. Recent advances in high performance blue organic light-emitting diodes based on fluorescence emitters. J. Mater. Chem. C 8 , 2614–2642 (2020).
Salehi, A. et al. Realization of high-efficiency fluorescent organic light-emitting diodes with low driving voltage. Nat. Commun. 10 , 2305 (2019).
Yoshida, K. et al. 245 MHz bandwidth organic light-emitting diodes used in a gigabit optical wireless data link. Nat. Commun. 11 , 1171 (2020).
Fukuda, T., Okada, T., Wei, B., Ichikawa, M. & Taniguchi, Y. Transient property of optically pumped organic film of different fluorescence lifetimes. Appl. Phys. Lett. 90 , 1–4 (2007).
Chime, A. C. et al. Electrical modelling and design of ultra-fast micro-OLED with coplanar wave-guided electrodes in ON–OFF regime. Org. Electron. 56 , 284–290 (2018).
Ruhstaller, B. et al. Transient and steady-state behavior of space charges in multilayer organic light-emitting diodes. J. Appl. Phys. 89 , 4575–4586 (2001).
Xiao, X. et al. Improving the modulation bandwidth of LED by CdSe/ZnS quantum dots for visible light communication. Opt. Express 24 , 21577 (2016).
Dursun, I. et al. Perovskite nanocrystals as a color converter for visible light communication. ACS Photon. 3 , 1150–1156 (2016).
Kang, C. H. et al. High-speed colour-converting photodetector with all-inorganic CsPbBr3 perovskite nanocrystals for ultraviolet light communication. Light Sci. Appl. 8 , 2047–7538 (2019).
Xiao, H. et al. Effects of injection current on the modulation bandwidths of quantum-dot light-emitting diodes. IEEE Trans. Electron Devices 66 , 4805–4810 (2019).
Xiao, H. et al. 4 Mb/s under a 3 m transmission distance using a quantum dot light-emitting diode and NRZ-OOK modulation. Opt. Lett. 45 , 1297 (2020).
Xiao, H., Wang, R., Wang, K., Chen, W. & Chiang, K. S. Trade-offs between illumination and modulation performances of quantum-dot LED. IEEE Photon. Technol. Lett. 32 , 726–729 (2020).
Gong, X. et al. Highly efficient quantum dot near-infrared light-emitting diodes. Nat. Photon. 10 , 253–257 (2016).
Pu, C. et al. Electrochemically-stable ligands bridge the photoluminescence-electroluminescence gap of quantum dots. Nat. Commun. 11 , 937 (2020).
Deng, W. et al. 2D Ruddlesden–Popper perovskite nanoplate based deep‐blue light‐emitting diodes for light communication. Adv. Funct. Mater. 29 , 1903861 (2019).
Bao, C. et al. Bidirectional optical signal transmission between two identical devices using perovskite diodes. Nat. Electron. 3 , 156–164 (2020).
Xu, W. et al. Rational molecular passivation for high-performance perovskite light-emitting diodes. Nat. Photon. 13 , 418–424 (2019).
Zou, W. et al. Minimising efficiency roll-off in high-brightness perovskite light-emitting diodes. Nat. Commun. 9 , 608 (2018).
Zhao, L. et al. Thermal management enables bright and stable perovskite light-emitting diodes. Adv. Mater. 32 , 2000752 (2020).
Friend, R. H. et al. Electroluminescence in conjugated polymers. Nature 397 , 121–128 (1999).
Baldo, M. A. et al. Highly efficient phosphorescent emission from organic electroluminescent devices. Nature 395 , 151–154 (1998).
O’Brien, D. F., Baldo, M. A., Thompson, M. E. & Forrest, S. R. Improved energy transfer in electrophosphorescent devices. Appl. Phys. Lett. 74 , 442–444 (1999).
Shin, H. et al. Sky-blue phosphorescent OLEDs with 34.1% external quantum efficiency using a low refractive index electron transporting layer. Adv. Mater. 28 , 4920–4925 (2016).
Lu, G. Z. et al. Rapid room temperature synthesis of red iridium(III) complexes containing a four-membered Ir–S–C–S chelating ring for highly efficient OLEDs with EQE over 30%. Chem. Sci. 10 , 3535–3542 (2019).
Sinha, S., Rothe, C., Güntner, R., Scherf, U. & Monkman, A. P. Electrophosphorescence and delayed electroluminescence from pristine polyfluorene thin-film devices at low temperature. Phys. Rev. Lett. 90 , 127402 (2003).
Endo, A. et al. Thermally activated delayed fluorescence from Sn 4+ –porphyrin complexes and their application to organic light-emitting diodes—a novel mechanism for electroluminescence. Adv. Mater. 21 , 4802–4806 (2009).
Uoyama, H., Goushi, K., Shizu, K., Nomura, H. & Adachi, C. Highly efficient organic light-emitting diodes from delayed fluorescence. Nature 492 , 234–238 (2012).
Goushi, K., Yoshida, K., Sato, K. & Adachi, C. Organic light-emitting diodes employing efficient reverse intersystem crossing for triplet-to-singlet state conversion. Nat. Photon. 6 , 253–258 (2012).
Li, W. et al. A twisting donor-acceptor molecule with an intercrossed excited state for highly efficient, deep-blue electroluminescence. Adv. Funct. Mater. 22 , 2797–2803 (2012).
Di, D. et al. High-performance light-emitting diodes based on carbene-metal-amides. Science 356 , 159–163 (2017).
Hall, C. R., Romanov, A. S., Bochmann, M. & Meech, S. R. Ultrafast structure and dynamics in the thermally activated delayed fluorescence of a carbene-metal-amide. J. Phys. Chem. Lett. 9 , 5873–5876 (2018).
Xiang, C., Peng, C., Chen, Y. & So, F. Origin of sub-bandgap electroluminescence in organic light-emitting diodes. Small 11 , 5439–5443 (2015).
Ai, X. et al. Efficient radical-based light-emitting diodes with doublet emission. Nature 563 , 536–540 (2018).
Abdurahman, A. et al. Understanding the luminescent nature of organic radicals for efficient doublet emitters and pure-red light-emitting diodes. Nat. Mater. 19 , 1224–1229 (2020).
Shang, Y. & Ning, Z. Colloidal quantum-dots surface and device structure engineering for high-performance light-emitting diodes. Natl Sci. Rev. 4 , 170–183 (2017).
Chen, F., Guan, Z. & Tang, A. Nanostructure and device architecture engineering for high-performance quantum-dot light-emitting diodes. J. Mater. Chem. C 6 , 10958–10981 (2018).
Pal, B. N. et al. ‘Giant’ CdSe/CdS core/shell nanocrystal quantum dots as efficient electroluminescent materials: Strong influence of shell thickness on light-emitting diode performance. Nano Lett. 12 , 331–336 (2012).
Bae, W. K. et al. Controlled alloying of the core-shell interface in CdSe/CdS quantum dots for suppression of auger recombination. ACS Nano 7 , 3411–3419 (2013).
Yang, Y. et al. High-efficiency light-emitting devices based on quantum dots with tailored nanostructures. Nat. Photon. 9 , 259–265 (2015).
Peng, X., Schlamp, M. C., Kadavanich, A. V. & Alivisatos, A. P. Epitaxial growth of highly luminescent CdSe/CdS core/shell nanocrystals with photostability and electronic accessibility. J. Am. Chem. Soc. 119 , 7019–7029 (1997).
Murray, C. B., Norris, D. J. & Bawendi, M. G. Synthesis and characterization of nearly monodisperse CdE (E = S, Se, Te) semiconductor nanocrystallites. J. Am. Chem. Soc. 115 , 8706–8715 (1993).
Shrestha, A., Batmunkh, M., Tricoli, A., Qiao, S. Z. & Dai, S. Near-infrared active lead chalcogenide quantum dots: preparation, post-synthesis ligand exchange, and applications in solar cells. Angew. Chem. Int. Ed. 58 , 5202–5224 (2019).
Shen, H. et al. Visible quantum dot light-emitting diodes with simultaneous high brightness and efficiency. Nat. Photon. 13 , 192–197 (2019).
Kagan, C. R., Murray, C. B., Nirmal, M. & Bawendi, M. G. Electronic energy transfer in CdSe quantum dot solids. Phys. Rev. Lett. 76 , 1517–1520 (1996).
Pradhan, S. et al. High-efficiency colloidal quantum dot infrared light-emitting diodes via engineering at the supra-nanocrystalline level. Nat. Nanotechnol. 14 , 72–79 (2019).
Gao, L. et al. Efficient near-infrared light-emitting diodes based on quantum dots in layered perovskite. Nat. Photon. 14 , 227–233 (2020).
Vasilopoulou, M. et al. Efficient colloidal quantum dot light-emitting diodes operating in the second near-infrared biological window. Nat. Photon. 14 , 50–56 (2020).
Yuan, Z. et al. Unveiling the synergistic effect of precursor stoichiometry and interfacial reactions for perovskite light-emitting diodes. Nat. Commun. 10 , 2818 (2019).
Miao, Y. et al. Stable and bright formamidinium-based perovskite light-emitting diodes with high energy conversion efficiency. Nat. Commun. 10 , 1–7 (2019).
Cho, H. et al. Overcoming the electroluminescence efficiency limitations of perovskite light-emitting diodes. Science 350 , 1222–1225 (2015).
Zheng, K. et al. Exciton binding energy and the nature of emissive states in organometal halide perovskites. J. Phys. Chem. Lett. 6 , 2969–2975 (2015).
Stranks, S. D., Hoye, R. L. Z., Di, D., Friend, R. H. & Deschler, F. The physics of light emission in halide perovskite devices. Adv. Mater. 31 , 1803336 (2019).
Schmidt, L. C. et al. Nontemplate synthesis of CH 3 NH 3 PbBr 3 perovskite nanoparticles. J. Am. Chem. Soc. 136 , 850–853 (2014).
Protesescu, L. et al. Nanocrystals of cesium lead halide perovskites (CsPbX 3 , X = Cl, Br, and I): novel optoelectronic materials showing bright emission with wide color gamut. Nano Lett. 15 , 3692–3696 (2015).
Zhang, F. et al. Brightly luminescent and color-tunable colloidal CH 3 NH 3 PbX 3 (X = Br, I, Cl) quantum dots: potential alternatives for display technology. ACS Nano 9 , 4533–4542 (2015).
Wang, H. et al. Perovskite-molecule composite thin films for efficient and stable light-emitting diodes. Nat. Commun. 11 , 891 (2020).
Hong, X., Ishihara, T. & Nurmikko, A. V. Dielectric confinement effect on excitons in PbI 4 -based layered semiconductors. Phys. Rev. B 45 , 6961–6964 (1992).
Yuan, M. et al. Perovskite energy funnels for efficient light-emitting diodes. Nat. Nanotechnol. 11 , 872–877 (2016).
Wang, N. et al. Perovskite light-emitting diodes based on solution-processed self-organized multiple quantum wells. Nat. Photon. 10 , 699–704 (2016).
Shang, Y. et al. Highly stable hybrid perovskite light-emitting diodes based on Dion–Jacobson structure. Sci. Adv. 5 , eaaw8072 (2019).
Yang, X. et al. Efficient green light-emitting diodes based on quasi-two-dimensional composition and phase engineered perovskite with surface passivation. Nat. Commun. 9 , 570 (2018).
Wang, Q., Ren, J., Peng, X. F., Ji, X. X. & Yang, X. H. Efficient sky-blue perovskite light-emitting devices based on ethylammonium bromide induced layered perovskites. ACS Appl. Mater. Interfaces 9 , 29901–29906 (2017).
Dong, Y. et al. Bipolar-shell resurfacing for blue LEDs based on strongly confined perovskite quantum dots. Nat. Nanotechnol. 15 , 668–674 (2020).
Matsushima, T. et al. High performance from extraordinarily thick organic light-emitting diodes. Nature 572 , 502–506 (2019).
Wang, Z. et al. Manipulating the trade-off between quantum yield and electrical conductivity for high-brightness quasi-2D perovskite light-emitting diodes. Adv. Funct. Mater. 28 , 1804187 (2018).
Adachi, C., Baldo, M. A., Thompson, M. E. & Forrest, S. R. Nearly 100% internal phosphorescence efficiency in an organic light emitting device. J. Appl. Phys. 90 , 5048–5051 (2001).
Richter, J. M. et al. Enhancing photoluminescence yields in lead halide perovskites by photon recycling and light out-coupling. Nat. Commun. 7 , 1341 (2016).
Chen, Z. et al. Utilization of trapped optical modes for white perovskite light-emitting diodes with efficiency over 12%. Joule 5 , 456–466 (2021).
Sun, Y. & Forrest, S. R. Enhanced light out-coupling of organic light-emitting devices using embedded low-index grids. Nat. Photon. 2 , 483–487 (2008).
Koo, W. H. et al. Light extraction from organic light-emitting diodes enhanced by spontaneously formed buckles. Nat. Photon. 4 , 222–226 (2010).
Dodabalapur, A., Rothberg, L. J., Miller, T. M. & Kwock, E. W. Microcavity effects in organic semiconductors. Appl. Phys. Lett. 64 , 2486–2488 (1994).
Ramuz, M., Bürgi, L., Stanley, R. & Winnewisser, C. Coupling light from an organic light emitting diode (OLED) into a single-mode waveguide: toward monolithically integrated optical sensors. J. Appl. Phys. 105 , 184508 (2009).
Ratcliff, E. L. et al. A planar, chip-based, dual-beam refractometer using an integrated organic light-emitting diode (OLED) light source and organic photovoltaic (OPV) detectors. Anal. Chem. 82 , 2734–2742 (2010).
Haigh, P. A., Ghassemlooy, Z., Rajbhandari, S. & Papakonstantinou, I. Visible light communications using organic light emitting diodes. IEEE Commun. Mag. 51 , 148–154 (2013).
Rajbhandari, S. et al. A review of gallium nitride LEDs for multi-gigabit-per-second visible light data communications. Semicond. Sci. Technol. 32 , 023001 (2017).
Schubert, E. F., Gessmann, T. & Kim, J. K. Organic Light Emitting Devices: Synthesis, Properties and Applications (eds Müllen, K. & Scherf, U.) 1–33 (Wiley, 2005); https://doi.org/10.1002/3527607986.ch1
Wang, Z. et al. Warm-white-light-emitting diode based on a dye-loaded metal–organic framework for fast white-light communication. ACS Appl. Mater. Interfaces 9 , 35253–35259 (2017).
Khalid, A. M., Cossu, G., Corsini, R., Choudhury, P. & Ciaramella, E. 1-Gb/s transmission over a phosphorescent white LED by using rate-adaptive discrete multitone modulation. IEEE Photon. J. 4 , 1465–1473 (2012).
Huang, X., Wang, Z., Shi, J., Wang, Y. & Chi, N. 16 Gbit/s phosphorescent white LED based VLC transmission using a cascaded pre-equalization circuit and a differential outputs PIN receiver. Opt. Express 23 , 22034 (2015).
Manousiadis, P. P., Yoshida, K., Turnbull, G. A. & Samuel, I. D. W. Organic semiconductors for visible light communications. Philos. Trans. R. Soc. A 378 , 20190186 (2020).
Chun, H. et al. Visible light communication using a blue GaN μ LED and fluorescent polymer color converter. IEEE Photon. Technol. Lett. 26 , 2035–2038 (2014).
Sajjad, M. T. et al. Novel fast color-converter for visible light communication using a blend of conjugated polymers. ACS Photon. 2 , 194–199 (2015).
Vithanage, D. A. et al. BODIPY star-shaped molecules as solid state colour converters for visible light communications. Appl. Phys. Lett. 109 , 013302 (2016).
Zhang, Y. et al. Aggregation-induced emission luminogens as color converters for visible-light communication. ACS Appl. Mater. Interfaces 10 , 34418–34426 (2018).
Mei, S. et al. High-bandwidth white-light system combining a micro-LED with perovskite quantum dots for visible light communication. ACS Appl. Mater. Interfaces 10 , 5641–5648 (2018).
Zhou, Z. et al. Hydrogen peroxide-treated carbon dot phosphor with a bathochromic-shifted, aggregation-enhanced emission for light-emitting devices and visible light communication. Adv. Sci. 5 , 1800369 (2018).
Liu, E. et al. Highly emissive carbon dots in solid state and their applications in light-emitting devices and visible light communication. ACS Sustain. Chem. Eng. 7 , 9301–9308 (2019).
Geffroy, B., le Roy, P. & Prat, C. Organic light-emitting diode (OLED) technology: materials, devices and display technologies. Polym. Int. 55 , 572–582 (2006).
Zhang, H., Chen, S. & Sun, X. W. Efficient red/green/blue tandem quantum-dot light-emitting diodes with external quantum efficiency exceeding 21%. ACS Nano 12 , 697–704 (2018).
Lee, K. H. et al. Highly efficient, color-reproducible full-color electroluminescent devices based on red/green/blue quantum dot-mixed multilayer. ACS Nano 9 , 10941–10949 (2015).
Luo, J. et al. Efficient and stable emission of warm-white light from lead-free halide double perovskites. Nature 563 , 541–545 (2018).
Zou, S. et al. Stabilizing cesium lead halide perovskite lattice through Mn(II) substitution for air-stable light-emitting diodes. J. Am. Chem. Soc. 139 , 11443–11450 (2017).
Euvrard, J., Yan, Y. & Mitzi, D. B. Electrical doping in halide perovskites. Nat. Rev. Mater. 6 , 531–549 (2021).
Poddubny, A., Iorsh, I., Belov, P. & Kivshar, Y. Hyperbolic metamaterials. Nat. Photon. 7 , 958–967 (2013).
Hou, X. et al. Engineering Auger recombination in colloidal quantum dots via dielectric screening. Nat. Commun. 10 , 1750 (2019).
Wu, Z., Liu, P., Zhang, W., Wang, K. & Sun, X. W. Development of InP quantum dot-based light-emitting diodes. ACS Energy Lett. 5 , 1095–1106 (2020).
Jiang, C. et al. Printed subthreshold organic transistors operating at high gain and ultralow power. Science 363 , 719–723 (2019).
Zhang, C. et al. Organic printed photonics: from microring lasers to integrated circuits. Sci. Adv. 1 , e1500257 (2015).
Lee, M. et al. Broadband modulation of light by using an electro-optic polymer. Science 298 , 1401–1403 (2002).
Zhu, M. et al. Optical single side-band Nyquist PAM-4 transmission using dual-drive MZM modulation and direct detection. Opt. Express 26 , 6629 (2018).
Fan, Q., Zhou, G., Gui, T., Lu, C. & Lau, A. P. T. Advancing theoretical understanding and practical performance of signal processing for nonlinear optical communications through machine learning. Nat. Commun. 11 , 3694 (2020).
Zhang, Y., Wang, L., Wang, K., Wong, K. S. & Wu, K. Recent advances in the hardware of visible light communication. IEEE Access 7 , 91093–91104 (2019).
Agrawal, G. P. Fiber ‐ Optic Communication Systems (Wiley, 2010); https://doi.org/10.1002/9780470918524
Download references
Acknowledgements
We thank X. Li for valuable discussions and arguments. H.W. thanks D. Dong for the screen bar that helped when drafting this article. This work was supported by EPSRC (2015; EP/M015165/1), the National Natural Science Foundation of China (61974014), the National Key Research and Development Program of China (2019YFB2203400), the ‘111 Project’ (B20030), the Fundamental Research Funds for the Central Universities (ZYGX2019Z018), the Innovation Group Project of Sichuan Province (20CXTD0090) and the UESTC Shared Research Facilities of Electromagnetic Wave and Matter Interaction (Y0301901290100201). W.Z. thanks the financial support from EPSRC New Investigator Award (2018; EP/R043272/1) and H2020-EU grant (2018; CORNET 760949).
Author information
These authors contributed equally: Aobo Ren, Hao Wang.
Authors and Affiliations
Institute of Fundamental and Frontier Sciences, University of Electronic Science and Technology of China, Chengdu, China
Aobo Ren, Jiang Wu & Zhiming Wang
Advanced Technology Institute, University of Surrey, Guildford, UK
Aobo Ren & Wei Zhang
Centre for Photonic Systems, Department of Engineering, University of Cambridge, Cambridge, UK
Hao Wang, Richard V. Penty & Ian H. White
State Key Laboratory of Electronic Thin Films and Integrated Devices, University of Electronic Science and Technology of China, Chengdu, China
Vice-Chancellor’s Office, University of Bath, Bath, UK
Ian H. White
You can also search for this author in PubMed Google Scholar
Contributions
All authors conceived this work and contributed to the discussion of content. A.R. and H.W. researched the data and wrote the first draft. W.Z., J.W. and I.H.W. revised the manuscript before submission.
Corresponding authors
Correspondence to Wei Zhang or Jiang Wu .
Ethics declarations
Competing interests.
The authors declare no competing interests.
Additional information
Peer review information Nature Electronics thanks Jiang Tang, Lina Quan and the other, anonymous, reviewer(s) for their contribution to the peer review of this work.
Publisher’s note Springer Nature remains neutral with regard to jurisdictional claims in published maps and institutional affiliations.

Rights and permissions
Reprints and permissions
About this article
Cite this article.
Ren, A., Wang, H., Zhang, W. et al. Emerging light-emitting diodes for next-generation data communications. Nat Electron 4 , 559–572 (2021). https://doi.org/10.1038/s41928-021-00624-7
Download citation
Received : 08 May 2020
Accepted : 25 June 2021
Published : 23 August 2021
Issue Date : August 2021
DOI : https://doi.org/10.1038/s41928-021-00624-7
Share this article
Anyone you share the following link with will be able to read this content:
Sorry, a shareable link is not currently available for this article.
Provided by the Springer Nature SharedIt content-sharing initiative
This article is cited by
Frequency-selective perovskite photodetector for anti-interference optical communications.
- Liangliang Min
- Haoxuan Sun
Nature Communications (2024)
A three-terminal light emitting and detecting diode
- Muhammad Hunain Memon
- Haiding Sun
Nature Electronics (2024)
Switchable interfacial reaction enables bright and stable deep-red perovskite light-emitting diodes
- Jiejun Zeng
- Xiaoyue Sun
- Yizheng Jin
Nature Photonics (2024)
Electrical control of excitonic oscillator strength and spatial distribution in a monolayer semiconductor
- Yanming Wang
- Junrong Zhang
- Junyong Wang
Nano Research (2024)
NHC-derived carbon-centered luminescent radicals with short-wavelength emission via suppression of Kasha’s rule
- Ying-Feng Han
Science China Chemistry (2024)
Quick links
- Explore articles by subject
- Guide to authors
- Editorial policies
Sign up for the Nature Briefing newsletter — what matters in science, free to your inbox daily.


An official website of the United States government
The .gov means it’s official. Federal government websites often end in .gov or .mil. Before sharing sensitive information, make sure you’re on a federal government site.
The site is secure. The https:// ensures that you are connecting to the official website and that any information you provide is encrypted and transmitted securely.
- Publications
- Account settings
Preview improvements coming to the PMC website in October 2024. Learn More or Try it out now .
- Advanced Search
- Journal List
- Photomed Laser Surg
A Controlled Trial to Determine the Efficacy of Red and Near-Infrared Light Treatment in Patient Satisfaction, Reduction of Fine Lines, Wrinkles, Skin Roughness, and Intradermal Collagen Density Increase
Alexander wunsch.
1 Medical Light Consulting, Heidelberg, Germany.
Karsten Matuschka
2 JK-International GmbH, Windhagen, Germany.
Objective: The purpose of this study was to investigate the safety and efficacy of two novel light sources for large area and full body application, providing polychromatic, non-thermal photobiomodulation (PBM) for improving skin feeling and appearance. Background data: For non-thermal photorejuvenation, laser and LED light sources have been demonstrated to be safe and effective. However, lasers and LEDs may offer some disadvantages because of dot-shaped (punctiform) emission characteristics and their narrow spectral bandwidths. Because the action spectra for tissue regeneration and repair consist of more than one wavelength, we investigated if it is favorable to apply a polychromatic spectrum covering a broader spectral region for skin rejuvenation and repair. Materials and methods: A total of 136 volunteers participated in this prospective, randomized, and controlled study. Of these volunteers, 113 subjects randomly assigned into four treatment groups were treated twice a week with either 611–650 or 570–850 nm polychromatic light (normalized to ∼9 J/cm 2 in the range of 611–650 nm) and were compared with controls ( n =23). Irradiances and treatment durations varied in all treatment groups. The data collected at baseline and after 30 sessions included blinded evaluations of clinical photography, ultrasonographic collagen density measurements, computerized digital profilometry, and an assessment of patient satisfaction. Results: The treated subjects experienced significantly improved skin complexion and skin feeling, profilometrically assessed skin roughness, and ultrasonographically measured collagen density. The blinded clinical evaluation of photographs confirmed significant improvement in the intervention groups compared with the control. Conclusions: Broadband polychromatic PBM showed no advantage over the red-light-only spectrum. However, both novel light sources that have not been previously used for PBM have demonstrated efficacy and safety for skin rejuvenation and intradermal collagen increase when compared with controls.
Introduction
A ltering cellular function using low level, non-thermal LED light is called photobiomodulation (PBM) or low-level light therapy (LLLT), and is a medical treatment modality of increasing clinical importance. 1 Because of the combination of high degree of penetration in skin 2 and absorption by respiratory chain components, light in the spectral range from 600 to 1300 nm is useful for promoting wound healing, tissue repair, and skin rejuvenation. 3–5 In contrast to traumatic ablative (e.g., laser resurfacing) and non-ablative (e.g., intense pulsed light [IPL]) skin rejuvenation modalities that induce secondary tissue repair by causing controlled damage to either the epidermis or the dermis, PBM is atraumatic, and bypasses the initial destructive step by directly stimulating regenerative processes in the skin. Its action mechanisms encompass increased cellular proliferation, migration, and adhesion. 6 Important cell types for skin and tissue regeneration are fibroblasts, keratinocytes, and immune cells (mast cells, neutrophils, and macrophages), which can be stimulated using specific wavelengths with significant tissue penetration properties. 7 The known severe side effects of traumatic skin rejuvenation procedures, such as inflammation, unpleasant pain perception, and prolonged social down time, 8 are unknown in PBM; PBM has been successfully administered to reduce common symptoms of laser resurfacing and IPL treatment. 9 Photon emitters, such as lasers or LEDs, have proven to be effective light sources for PBM during recent decades, thereby demonstrating that it is not the technical type of light source but the treatment parameters such as wavelength, irradiance, and fluence that are likely to be accountable for the effects. 10 However, laser and LED light sources may offer some disadvantages because of their dot-shaped (punctiform) emission characteristics and narrow spectral bandwidths. Because the action spectra for tissue regeneration and repair consist of more than one wavelength, 7 , 11 it might be favorable to apply a polychromatic spectrum covering a broader spectral region for skin rejuvenation and skin repair. We investigated the safety and efficacy of a novel non-thermal, non-ablative, atraumatic, polychromatic low-level light treatment modality with a focus on pleasant skin feeling, improved skin appearance, intradermal collagen increase, and the visible reduction of fine lines and wrinkles in a prospective, randomized, controlled trial that consisted of 136 volunteers.
Materials and Methods
Study population and design.
We conducted a randomized, controlled clinical trial between January 2012 and December 2012. Table 1 summarizes the baseline (t0) characteristics of the subject groups.
Baseline (t0) Characteristics of the Subject Groups
n= | n= | n= | |
---|---|---|---|
Sex | |||
Female | 49/86.0% | 34/70.8% | 15/65.2% |
Male | 8/14.0% | 14/29.2% | 8/34.8% |
Age | 46.2±9.0 | 48.6±9.8 | 44.4±10.2 |
Weight | 72.9±15.22 | 73.4±13.7 | 73.7±13.4 |
Skin complexion (subjective) | 4.54±1.92 | 4.87±2.02 | |
Skin feeling (subjective) | 5.33±2.04 | 5.24±2.18 | |
Skin roughness (R ) | 15.29±4.20 | 14.84±4.04 | 11.79±2.17 |
Collagen intensity score | 20.40±6.55 | 18.96±3.54 | 23.22±7.36 |
Expert wrinkle assessment | |||
No/shallow or fine wrinkles | 14/24.6% | 17/35.4% | 5/21.7% |
Moderate wrinkles | 20/35.1% | 11/22.9% | 6/26.1% |
Prominent or deep wrinkles | 13/22.8% | 11/22.9% | 9/39.1% |
No majority vote possible | 10/17.5% | 9/18.8% | 3/13.0% |
RLT, red light technology; ELT, energizing light technology.
The subjects were between 27 and 79 years of age. Inclusion criteria were the capacity to independently position oneself to use the device, the capacity to understand the treatment, a signed declaration of consent, and interest in continuous participation. The exclusion criteria were physical and psychological disease casting doubt on the capacity to consent, preliminary treatment with red light within the 6 months prior to the beginning of the study, recent invasive cosmetic procedures such as Botox during the 12 months prior to the beginning of the study, acute or prior skin cancer, acute skin disease requiring dermatological treatment, existing or planned pregnancy, lactation, history of photosensitivity or recent use of photosensitizing medication, epilepsy, and the tendency to faint. All of the participants gave written informed consent for this study, which was approved by the Ethics Committee of the Medical Association (Landesärztekammer) Baden-Württemberg, Stuttgart, Germany. The investigation was conducted in accordance with the Declaration of Helsinki (DoH/Oct2008). After the declaration of informed consent following examination of the inclusion and exclusion criteria, each participant was assigned to one of four groups using a computerized randomization process. Group 5 was mainly recruited from employees of the JK company without randomization, and served as the control. Groups 1–4 were treated twice a week with 30 treatments in total, starting in January 2012. To minimize the influence of seasonal changes, the time interval for data acquisition at the baseline, t15, t30, and follow-up examinations was restricted to 1 month. The data acquisition at baseline was completed in February 2012, and all of the volunteers finished treatment 30 (t30) in June 2012.
The control group did not receive any treatment, as the therapy cannot be blinded, and a sham light source without any effect most likely does not exist. The control group volunteers participated in the clinical measurements only, and the acquisition of subjective parameters such as skin feeling and skin complexion was not conducted. Because of the similar spectral lamp characteristics for groups 1 and 2 and groups 3 and 4, groups 1 and 2 were combined for evaluation as the “mid-pressure lamp group” [energizing light technology (ELT)], and groups 3 and 4 were evaluated together as the “low-pressure lamp group” [red light technology (RLT)] to obtain larger group sizes and, therefore, higher statistical power. Nevertheless, the subdivision into groups 1–4 allowed us to compare outcomes based on different treatment parameters, such as spectral distribution, irradiance, and fluence. A questionnaire concerning the tolerability of the application was filled in after each treatment (t1–t30). Digital photographs and clinical measurements were taken, and subjective questionnaires were used to assess complexion and skin feeling at the baseline (t0) and after 15 (t15) and 30 treatments (t30). The follow-up acquisition of subjective and clinical parameters was conducted at t30+6 months.
Light Sources
Four units equipped with two different types of polychromatic light sources (low-pressure vs. mid-pressure lamps) were used to conduct this study. Table 2 lists the lamp technologies, lamp types, treatment area (full or part of the body), spectral values, session duration, and treatment doses for the units used in this study.
Characteristics of the Treatment Units, Light Sources, and Application Parameters
Technology | Energizing light (ELT) | Energizing light (ELT) | Red light (RLT) | Red light (RLT) |
Lamp type | Medium pressure | Medium pressure | Low pressure | Low pressure |
Treatment area | Partial-body | Full-body | Full-body | Full-body |
Treatment position | Semi-reclined | Horizontal | Horizontal | Vertical |
Irradiance (611–650 nm) | 7.1 mW/cm | 10.4 mW/cm | 5.9 mW/cm | 13.3 mW/cm |
Total irradiance (570–850 nm) | 42.8 mW/cm | 54.8 mW/cm | 10.3 mW/cm | 23.4 mW/cm |
Treatment duration | 20 min | 15 min | 25 min | 12 min |
Treatment dose (611–650 nm) | 8.5 J/cm | 9.4 J/cm | 8.9 J/cm | 9.6 J/cm |
Total radiant exposure (570–850 nm) | 51.4 J/cm | 49.3 J/cm | 15.5 J/cm | 16.8 J/cm |
Treatment units 2, 3, and 4 provided full-body irradiation, covering the ventral and dorsal surfaces of the head, neck, trunk, upper limbs, and lower limbs at the same time. Full-body irradiation units 2 and 3 enabled treatment with the patient in a horizontal, reclined position, whereas unit 4 was engineered as a cabin for vertical treatment orientation. Unit 1 was designed for the local treatment of the face and décolletage area with the patient sitting in a chair in a semi-reclined position. Units 1 and 2 were equipped with medium-pressure gas discharge lamps in combination with spectrally selective reflectors and corresponding filter systems, to eliminate spectral emissions in wavelengths <570 and >850 nm; these units were denoted as ELT. Units 3 and 4 were equipped with low-pressure gas discharge fluorescent lamp tubes providing a spectral emission peak predominantly within the range of 611–650 nm, denoted as RLT. Because of the different spectral properties and irradiances, we defined the spectral range between 611 and 650 nm for the calculation of treatment fluences. This wavelength window encompasses 632.8 nm, which is a paramount wavelength in LLLT and PBM, representing the dominant wavelength of a HeNe-laser. The spectral dose distributions of the ELT and RLT light sources are shown in Fig. 1 , with the doses of both light sources normalized to 100 % for the 611–650 nm range. The treatment doses were kept constant for this spectral range, whereas irradiances and treatment durations varied for all four treatment groups in order to investigate the applicability of the Bunsen–Roscoe law of reciprocity within the given parametrical limits.

Spectral dose distributions of energizing light technology (ELT) and red light technology (RLT) light sources. Relationship between doses and wavelength ranges for ELT and RLT light sources, normalized to the spectral range 611–650 nm. Colored bars represent the spectral doses in percentages.
All units emitted almost no erythemogenic UV radiation (minimal erythema dose would not be reached after several hours of exposure, comparable to the UV emission of fluorescent lamps for general lighting service applications).
Measurements
The primary objective of the study was the improvement of subjective skin complexion and skin feeling. The volunteers were asked to specify their level of agreement to the statements in the questionnaire by marking a position along a continuous black line between two end points measuring 10 cm, which served as a visual analog scale (VAS). The secondary objectives were the improvement of measurement parameters using a DermaLab Combo (Cortex Technology, Hadsund, Denmark), a computer-supported skin diagnostics system equipped with a rotating high-resolution ultrasound sensor probe (20 MHz) for the determination of changes in intradermal collagen density, measured as a collagen intensity score (CIS). A Primos lite digital fringe projection system (GFM Messtechnik, Berlin, Germany) was used to measure the objective arithmetical roughness (R a ) of the skin surface in the periorbital region.
Photography
The digital photographs for the blinded wrinkle assessment were taken using a Nikon D5100 camera equipped with a Nikkor AF 50 mm 1:1.4 lens (Nikon Corporation, Chiyoda, Tokyo, Japan) and a Walimex RFL-3 ring light (Walser GmbH & Co. KG, Burgheim, Germany).
Subject outcome assessment
The subjective efficacy parameters were self-assessed at the baseline (t0), after 15 (t15) and 30 (t30) treatments, and after t30+6 months using 10 cm VAS for the improvements in skin complexion and skin feeling. These parameters were not assessed in the control group.
Objective clinical parameter assessment
The high-resolution ultrasound examination of collagen has enabled the measurement of visible changes in collagen density and numerical CISs representing the intradermal collagen fiber density. Profilometry yielded a numerical value for the R a of the skin area under examination.
Investigator assessment
Three independent physicians who were blinded to the clinical patient data, analyzed the clinical photographs obtained at t0 and t30. The investigators were instructed to arrange the randomly assorted sets of clinical photographs taken at t0 and t30 into a before/after treatment sequence. The baseline wrinkle depth according to the Modified Fitzpatrick Wrinkle Scale (MFWS) 12 and the degree of wrinkle reduction after treatment had to be assessed after sequencing. The votes of the investigators were summarized by the following majority rules: if two or three experts voted the same way, the agreed-upon classification was the summary measure; if all three experts voted differently, “no change” was the summary measure.
Statistical methods
The data in the tables are given as means±standard deviations. Comparisons of the changes in skin feeling, skin complexion, roughness, and collagen intensity from the baseline to t30 between the different treatment groups (intergroup comparisons) were performed using a linear model, with the baseline value of each volunteer as a covariate. Within-group differences from the baseline to values at t30 were assessed using the Mann–Whitney–Wilcoxon test. To compare wrinkle difference assessments among groups, we used the χ 2 test. Within groups, we tested the hypothesis of equal probabilities of improvement and worsening using binomial tests. All tests were two sided, and p values<0.05 were considered statistically significant.
Patient characteristics
Initially, 144 volunteers were recruited for the trial. Eight volunteers did not appear for the first appointment after randomization; therefore, the total number of patients finally included in the study was 136. Five volunteers stopped participating because of schedule incompatibilities and lack of time. One volunteer could not finish the treatment because of receiving antibiotic medication, which was one of the exclusion criteria; one volunteer terminated participation because of moving away; and one participant missed more than four treatments because of a period of residence at a health resort. Ultimately, 128 volunteers completed the treatment and the follow-up evaluation course, of whom 57 were treated with RLT, 48 were treated with ELT, and 23 were controls. The volunteers in the RLT and ELT groups were similar with respect to age, weight, skin complexion, skin feeling, skin roughness, and intradermal collagen density. The percentage of women was lower in the ELT group than in the RLT group. The controls had a slightly higher mean collagen density and a lower mean skin roughness.
Adverse events
None of the volunteers dropped out because of an adverse event. No severe adverse events were registered during the study or the follow-up phase. One volunteer with facial telangiectasia noticed an increased visibility after the first treatments, and decided to protect the zones in question from the light influence using a concealer for the rest of the treatment series. One volunteer experienced a reddening of scar tissue from a 40-year-old knee injury that was likely reactivated by the ELT 30 treatment. The affected scar healed completely within 1 week, and the treatments were continued without interruption.
Assessment of effects
Figure 2 shows two series of collagen ultrasonography scans, demonstrating the collagen density increase from t0 to t30 for one subject each in the RLT group and the ELT group.

Collagen ultrasonography examples.
Clinical photography revealed visible changes in wrinkles and skin roughness. Figure 3 shows an example for one subject in each treatment group, comparing the baseline (t0) status with t30.

Patient photography examples. (A) 64-year-old woman, energizing light technology (ELT). (B) 41-year-old woman, red light technology (RLT).
In Table 3 , the results of the t30−t0 measurements for each parameter in the different patient groups and the results of the expert wrinkle assessment are summarized. Within-group comparisons addressed whether the t30−t0 differences had means of zero for each patient group separately.
Comparison of the t30 − t0 Results Between and Within Subject Groups
n= | p | n= | p | n= | p | p | |
---|---|---|---|---|---|---|---|
Skin complexion (subjective) | −1.29±1.98 | <0.001 | −1.72±2.35 | <0.001 | 0.064 | ||
Skin feeling (subjective) | −1.01±2.30 | <0.001 | −1.65±2.17 | <0.001 | 0.167 | ||
Skin roughness (R ) | −1.79±2.46 | <0.001 | −1.58±2.22 | <0.001 | 0.95±1.45 | 0.003 | 0.003 |
Collagen intensity score | 5.75±4.54 | <0.001 | 6.40±5.17 | <0.001 | −0.26±5.09 | 0.84 | <0.001 |
Expert wrinkle assessment | <0.001 | <0.001 | <0.001 | <0.001 | |||
Better | 40/69% | 36/75% | 1/4% | ||||
Equal | 8/14% | 7/15% | 5/22% | ||||
Worse | 10/17% | 5/10% | 17/74% |
Analysis of covariance for the between-group comparison, one sample Wilcoxon-test for the within-group comparisons.
Within-group comparisons, t30−t0
In the RLT and ELT groups, skin complexion, skin feeling, collagen intensity score, skin roughness, and wrinkle status improved significantly ( p <0.001, Table 3 ). The skin feeling, skin complexion, and roughness changes were significantly ( p <0.001, covariance analysis) correlated with baseline values in all groups. In contrast, the control subjects showed no significant difference in collagen density and significant worsening of skin roughness and wrinkle status. These results are described in greater detail in Fig. 4 . Here, baseline measurements on the x-axis and the respective gain or reduction in the t30 values on the y-axis are color coded for the different treatment groups. In Fig. 4A, B and D , nearly all of the ELT and RLT points plotted below the baseline x-axis=0.00, indicating that the skin feeling, skin complexion, and roughness improved for nearly all of the volunteers ( p <0.01). In Fig. 4C (CIS), the baseline effect is not significant, whereas the CIS increase is significant ( p <0.001), and values above the x-axis indicate improvement.

Results for t30−t0. Changes t30−t0 (y-axis) are depicted in relation to the baseline value t0 on the x-axis. For A, B, and D, points below the x-axis indicate improvement; for C, points above the x-axis indicate improvement. The red light technology (RLT) and energizing light technology (ELT) t30 − t0 differences decrease with increasing baseline values.
Between-group comparisons
For the main efficacy parameters, skin complexion and skin feeling, we observed no significant differences between the RLT and ELT groups. The collagen density, roughness, and wrinkle status were significantly different among the three groups, as shown in Table 3 . There was no difference between the RLT and ELT groups, but there was a difference between both groups compared with controls, as shown by the blue points in Fig. 4C and D .
Subgroup analyses
We wanted to assess whether the two RLT treatment groups and the two ELT treatment groups showed different results; therefore, we compared the two groups. The RLT subgroups had 25 volunteers using CVT/RVT and 32 using C46 sun. There were no differences between the two groups with respect to skin complexion, skin feeling, skin roughness, collagen density, and wrinkle status. All of these parameters improved significantly between t0 and t30 (data not shown). We obtained very similar results for the two ELT groups, with 27 volunteers in ELT 30 and 21 volunteers in ELT 2.
The RLT group consisted of a lower percentage of male volunteers than did the ELT group and the control. Gender differences regarding the response to the PBM treatment for the main parameters were tested within each of the RLT/ELT/control subgroups using the Mann–Whitney U test, and we found no significant differences ( p >0.1 for all tests). Including gender as an additional covariate in the covariance analysis resulted in very similar p values for the tests regarding the comparison of study groups, compared with the analysis without gender. Only for collagen increase were gender and treatment both significant.
Long-term follow-up
The long-term results were analyzed for all subjects who were available for long-term follow-up in November/December 2012. A total of 52 of the 77 subjects who took part in the long-term follow-up finished after 30 treatments, 18 volunteers continued to a total of 45 treatments, and 7 volunteers received a total of 60 treatments (t60). To analyze the long-term effects, we tested whether the t60 measurements of skin feeling, skin complexion, CIS, and R a were better than the t0 measurements for the group of volunteers with 30 treatments. All volunteers had significantly better results at t60 (Wilcoxon test ≤0.001 for each). The t60−t0 differences were as follows: mean 0.99, SD 1.95 for skin feeling; mean −1.00, SD 2.10 for skin complexion; mean 5.10, SD 7.56 for CIS; and mean −0.64, SD 3.53 for R a . As expected, these differences displayed lower effect sizes than at t30. Only a group of seven volunteers continued the therapy with good results for a further 30 treatments, which may be partly the result of selection bias. Therefore, the long-term efficacy must be systematically evaluated in further studies. During the follow-up period, no delayed adverse events were recorded.
The use of LED light sources with 590, 633, and 830 nm wavelengths for athermal light-only photorejuvenation has grown rapidly in recent years. Additional wavelengths have been shown to be efficient in altering cellular functions, such as 570, 13 620, 680, 760, and 820 nm. 14 The treatment doses vary significantly, ranging from 0.1 J/cm 2 for 590 nm LED light with a specific sequence of pulsing, 15 up to 126 J/cm 2 for 633 nm continuous LED light. 16 , 17 The power of the light typically ranges between 1 and 1000 mW, depending upon the type of light source and the application. 1 Efficacy comparisons of the different devices available to the physician are not known to the authors.
This study is the first prospective clinical trial investigating the safety and efficacy of novel light sources for skin rejuvenation and the stimulation of dermal collagen synthesis based on low-pressure and mid-pressure gas discharge lamps. These light sources, in contrast to lasers and LEDs, allow simultaneous treatment with a tailored spectrum composed of several spectral bands that are effective in PBM. When compared with the initial values and the controls, the volunteers experienced significant improvements in their personal assessments of skin feeling and complexion, in clinical outcomes as assessed by collagen density and skin roughness measurements and in the reduction of fine lines and wrinkles as assessed by three blinded evaluators comparing t0 and t30 photographs.
Previous findings were able to correlate fibroblast activity and dermal matrix remodeling processes, with an increase in intradermal collagen density and reduced signs of aging. 18 The proposed underlying mechanisms include the photostimulation of terminal molecules in the electron transport chain and the subsequent adenosine triphosphate (ATP) concentration increase, 14 along with the selective light-driven activation of water molecules, 19 thereby enhancing metabolic exchange and influencing the ion transporter systems found in cellular membranes. 20 Detailed analysis of the gene expression profiles in human fibroblasts revealed an influence of low-intensity red light with a 628-nm wavelength on 111 different genes that are involved in cellular functions, such as cell proliferation; apoptosis; stress response; protein, lipid and carbohydrate metabolism; mitochondrial energy metabolism; DNA synthesis and repair; antioxidant related functions; and cytoskeleton- and cell-cell interaction-related functions. 21 A specific role of reactive oxygen species (ROS) in increasing fibroblast proliferation and motility has recently been reported, suggesting that the elevation of ROS via photodynamic therapy can enhance the cellular functions of dermal fibroblasts through specific mitogen-activated protein kinase (MAPK) signaling pathways in vitro . 22 The light-induced free radical formation in human skin has been investigated in detail, demonstrating that red light with 620 and 670 nm wavelengths increases the concentration of ROS even without the influence of external photosensitizers. 23
Because fibroblasts are responsible for collagen production in wound healing, dermal remodeling, and tissue repair, we decided to focus on increased collagen density as a surrogate marker for fibroblast activity, and abandoned such invasive monitoring methods as histologic examinations following skin biopsies for our study. Ultrasonographic collagen assessment is described as a feasible noninvasive methodology for monitoring dermal density during the senescence process. 24
A report of the stimulatory effects of 660 nm wavelength laser light on scar fibroblasts 25 could conceivably explain the potential reactivation of a>40-year-old knee injury, which occurred in one volunteer during the ELT treatment. Therefore, the influence of PBM on scar tissue should be subject to further investigation.
Some authors emphasize the importance of distinct wavelengths for optimal results. 16–18 , 26–28 In our study, the differences between the RLT and ELT treatments in clinical outcome and patient satisfaction were not significant, indicating that despite spectral differences, both light sources were commensurably effective regarding study objectives. Further studies of the treatment parameters are necessary.
The evaluation of clinical photography revealed a particular worsening of fine lines and wrinkles from t0 to t30 in the control group, which was not expected for a course of only 12 weeks. A possible explanation could be the seasonal variation of skin condition between winter and summer climates and the influence of solar radiation, as the clinical photography revealed skin pigmentation as a consequence of exposure to sunlight.
We observed a tendency that ELT/RLT treatment led to better results in female volunteers regarding the collagen density increase. This gender-specific response could conceivably be explained by physiological differences between male and female skin 29 , 30 on endocrine and extracellular matrix levels. However, gender-specific differences should be evaluated in greater detail in further investigations.
Conclusions
RLT and ELT are large-area and full-body treatment modalities for skin rejuvenation and improvements in skin feeling and skin complexion. The application of RLT and ELT provides a safe, non-ablative, non-thermal, atraumatic photobiomodulation treatment of skin tissue with high patient satisfaction rates. RLT and ELT can extend the spectrum of anti-aging treatment options available to patients looking for mild and pleasant light-only skin rejuvenation.
Acknowledgments
We thank Dr. Christine Fischer, Heidelberg, for help and advice regarding the statistical analysis of our data. We also thank all of the volunteers for their participation in this study. This study was fully funded by JK-Holding GmbH, Windhagen, Germany. All materials, light sources, and evaluation equipment were provided by the sponsor.
Author Disclosure Statement
The principal investigator (Alexander Wunsch) was mandated and remunerated by the sponsor to conduct the study. The authors have received funds to plan, conduct, and evaluate the study.
Accessibility Links
- Skip to content
- Skip to search IOPscience
- Skip to Journals list
- Accessibility help
- Accessibility Help
Click here to close this panel.
Purpose-led Publishing is a coalition of three not-for-profit publishers in the field of physical sciences: AIP Publishing, the American Physical Society and IOP Publishing.
Together, as publishers that will always put purpose above profit, we have defined a set of industry standards that underpin high-quality, ethical scholarly communications.
We are proudly declaring that science is our only shareholder.
A comparative study of fluorescent and LED lighting in industrial facilities
C Perdahci PhD 1 , H C Akin BSc 2 and O Cekic Msc 2
Published under licence by IOP Publishing Ltd IOP Conference Series: Earth and Environmental Science , Volume 154 , 2018 7th International Conference on Clean and Green Energy-ICCGE 2018 7–9 February 2018, Paris, France Citation C Perdahci PhD et al 2018 IOP Conf. Ser.: Earth Environ. Sci. 154 012010 DOI 10.1088/1755-1315/154/1/012010
Article metrics
1562 Total downloads
Share this article
Author affiliations.
1 Electrical Engineering Department, University of Kocaeli, Kocaeli, Turkey
2 Alkan Asia Africa Lighting Co., Fevzi Alkan Street, No:43 Sile, Turkey
Buy this article in print
Industrial facilities have always been in search for reducing outgoings and minimizing energy consumption. Rapid developments in lighting technology require more energy efficient solutions not only for industries but also for many sectors and for households. Addition of solid-state technology has brought LED lamps into play and with LED lamp usage, efficacy level has reached its current values. Lighting systems which uses fluorescent and LED lamps have become the prior choice for many industrial facilities. This paper presents a comparative study about fluorescent and LED based indoor lighting systems for a warehouse building in an industrial facility in terms of lighting distribution values, colour rendering, power consumption, energy efficiency and visual comfort. Both scenarios have been modelled and simulated by using Relux and photometric data for the luminaires have been gathered by conducting tests and measurements in an accredited laboratory.
Export citation and abstract BibTeX RIS
Content from this work may be used under the terms of the Creative Commons Attribution 3.0 licence . Any further distribution of this work must maintain attribution to the author(s) and the title of the work, journal citation and DOI.
IEEE Account
- Change Username/Password
- Update Address
Purchase Details
- Payment Options
- Order History
- View Purchased Documents
Profile Information
- Communications Preferences
- Profession and Education
- Technical Interests
- US & Canada: +1 800 678 4333
- Worldwide: +1 732 981 0060
- Contact & Support
- About IEEE Xplore
- Accessibility
- Terms of Use
- Nondiscrimination Policy
- Privacy & Opting Out of Cookies
A not-for-profit organization, IEEE is the world's largest technical professional organization dedicated to advancing technology for the benefit of humanity. © Copyright 2024 IEEE - All rights reserved. Use of this web site signifies your agreement to the terms and conditions.
Academia.edu no longer supports Internet Explorer.
To browse Academia.edu and the wider internet faster and more securely, please take a few seconds to upgrade your browser .
- We're Hiring!
- Help Center
LED Lighting
- Most Cited Papers
- Most Downloaded Papers
- Newest Papers
- Last »
- Zeppelin Follow Following
- Grecia Follow Following
- Aristoteles Follow Following
- Lighting Design Follow Following
- Architectural Lighting Follow Following
- Psychotherapy and Counseling Follow Following
- Power Engineering Follow Following
- Lighting Follow Following
- High Voltage Engineering Follow Following
- LED lighting design Follow Following
Enter the email address you signed up with and we'll email you a reset link.
- Academia.edu Journals
- We're Hiring!
- Help Center
- Find new research papers in:
- Health Sciences
- Earth Sciences
- Cognitive Science
- Mathematics
- Computer Science
- Academia ©2024

IMAGES
COMMENTS
Indeed, in the recent years, Light-Emitting Diodes (LEDs) have progressively made their way to the home lighting market, as well as to other mass markets. This article aims at giving an insight on LEDs physics, on the key inventions that led to the 2014 Nobel Prize and on the prospects for energy savings that LEDs could allow.
Because of the advent for LED as lighting device, LED lighting becomes one of the new trends in the lighting industry. In this paper, the power circuit design based on the constant current flyback ...
Light emitting diodes (LEDs) are solid-state devices that are highly energy efficient and considerably more durable compared with other forms of lighting, such as compact fluorescent lights (CFL) and traditional incandescents. LED technology has improved steadily over the years: every decade, the cost per lumen falls by a factor of 10 and the amount of light generated per LED package increases ...
This paper presents a survey of literature on the light (LEDs) as energy efficient light bulbs due to their high efficiency. The literature shows an increasing interest in this subject for the last decade, where the enhancement of LED lighting systems using various controllers has been widely investigated.
In approximately 63% of the papers dealing with light bulb technology, LEDs are compared with other light bulbs, or they are selected as the only illumination technology. ... Gentile, N., T. Goven, T. Laike, and K. Sjoberg. 2018. "A Field Study of Fluorescent and LED Classroom Lighting." Lighting Research & Technology 50: 631-650.
Ultraviolet light-emitting diodes based on group three nitrides 2008-Feb-01. The life and times of the LED — a 100-year history 2007-Apr-01. Nature Photonics Technology Focus: LEDs and their ...
Trade data showed that the United States imported $3.9 billion 12 ( Figure 2, right) in both traditional and LED luminaires. Market research revealed that the 2015 U.S. luminaire market was $17.3 billion, 4 which means that $13.4 billion (77%) of the luminaires in the U.S. market are manufactured domestically.
This paper presents a review of research on the failure mechanisms and failure modes of LEDs used in lighting systems. This paper provides a basis for understanding the reliability issues of LEDs ...
Carbon neutrality, energy savings, and lighting costs and quality have always led to urgent demand for lighting technology innovation. White light-emitting diodes (WLEDs) based on a single ...
6. Summary of Performance Parameters of Smart Street Lighting Research. Table 3 shows a summary of the performance parameters of some of the research work investigated in this paper. In summary, decentralized systems in which the dimming action is done on the light pole have a faster response as compared to centralized systems where the change ...
The invention of the blue light-emitting diode (LED) by Isamu Akasaki, Hiroshi Amano and Shuji Nakamura is the breakthrough for LED technology. Since then, LED has attracted wide attention from researchers who further investigate the performance of this technology. This study aims to identify research trends and determine future research on the LED using bibliometrics and network analysis. The ...
From research articles (Suntiti Yoomak et al., 2018, Suntiti Yoomak, 2018, Souza et al., 2019, Campisi et al., 2018, Dzombak et al., 2020, Gorgulu and Kocabey, 2020, Beccali et al., 2019), the use of LED luminaires overcomes not only the traditional light sources in public lighting systems in terms of energy saving, but also outstanding lighting quality, low maintenance, flexible light control ...
Lighting Research & Technology (LR&T) publishes original peer-reviewed research on all aspects of light and lighting and is published in association with The Society of Light and Lighting. LR&T covers the human response to light, the science of light generation, light control and measurement plus lighting design for both interior and exterior environments, as well as daylighting, energy ...
The paper outlines the concepts and design of an upcoming stand-alone solar photovoltaic system to supply the energy needs of a new proposed business complex. The purpose of this study is to ...
Keywords: renewable energies, LED lighting, wireless control, data monitoring, IoT-based devices, energy savings, sensors. INTRODUCTION Light Emitting Diode (LED) plays a fundamental role for energy saving and environmental protection in lighting industry. LED-based lights are up to 80% more efficient than traditional lighting sources such as
Organic light-emitting diodes (OLEDs) have rapidly grown as one of the leading technologies for full-color display panels and eco-friendly lighting sources due to their outstanding features including superior color quality, wide viewing angle, mercury-free manufacture, fascinating flexibility, etc. A variety 2020 Materials Chemistry Frontiers Review-type Articles
Fig. 7. Annual electricity cost of light-emitting diodes (LED) use (m −2) based on usage implied in research papers (see Supplementary Materials).Red, blue, green, and far-red may be given to replace (part of) baseline R:B or white light (see Fig. 6).Costs are based on 18 h·d −1 of 250 μmol·m −2 ·s −1 total PFD, with light treatments as described in Fig. 6.
performance that, in 1994, attain ed an astounding blue light luminous intensity more than 1 cd. Therefore, commercialized blue LED became possible. In recent d ecades, the technology of organic ...
Light-emitting diode (LED)-based communication links are of potential use in both free space and optical interconnect applications, and LEDs based on emerging semiconductor materials, which can ...
Introduction. A ltering cellular function using low level, non-thermal LED light is called photobiomodulation (PBM) or low-level light therapy (LLLT), and is a medical treatment modality of increasing clinical importance. 1 Because of the combination of high degree of penetration in skin 2 and absorption by respiratory chain components, light in the spectral range from 600 to 1300 nm is useful ...
This paper presents a comparative study about fluorescent and LED based indoor lighting systems for a warehouse building in an industrial facility in terms of lighting distribution values, colour rendering, power consumption, energy efficiency and visual comfort. Both scenarios have been modelled and simulated by using Relux and photometric ...
This paper presents the development of an intelligent system for controlling LED light intensity from the onsite and remote location. The objective of this paper is to save electric power by implementing the digitally controlled dimming of the LED light source from different intelligent methods. In order to implement the proposed scheme at onsite location, a microcontroller board is programmed ...
The performance of hemp seedlings was evaluated through morphological traits, photosynthetic pigments, and osmolytes under 11 light treatments (10 LED light compositions + natural light) in an aeroponics system. The seedlings were brought under treatment at 25 days of age, where the light intensity was 300 µmol m−2s−1 and duration was 20 days.
A research team led by the School of Engineering of the Hong Kong University of Science and Technology (HKUST) has developed full-color fiber light-emitting diodes utilizing perovskite quantum ...