Sciencing_Icons_Science SCIENCE
Sciencing_icons_biology biology, sciencing_icons_cells cells, sciencing_icons_molecular molecular, sciencing_icons_microorganisms microorganisms, sciencing_icons_genetics genetics, sciencing_icons_human body human body, sciencing_icons_ecology ecology, sciencing_icons_chemistry chemistry, sciencing_icons_atomic & molecular structure atomic & molecular structure, sciencing_icons_bonds bonds, sciencing_icons_reactions reactions, sciencing_icons_stoichiometry stoichiometry, sciencing_icons_solutions solutions, sciencing_icons_acids & bases acids & bases, sciencing_icons_thermodynamics thermodynamics, sciencing_icons_organic chemistry organic chemistry, sciencing_icons_physics physics, sciencing_icons_fundamentals-physics fundamentals, sciencing_icons_electronics electronics, sciencing_icons_waves waves, sciencing_icons_energy energy, sciencing_icons_fluid fluid, sciencing_icons_astronomy astronomy, sciencing_icons_geology geology, sciencing_icons_fundamentals-geology fundamentals, sciencing_icons_minerals & rocks minerals & rocks, sciencing_icons_earth scructure earth structure, sciencing_icons_fossils fossils, sciencing_icons_natural disasters natural disasters, sciencing_icons_nature nature, sciencing_icons_ecosystems ecosystems, sciencing_icons_environment environment, sciencing_icons_insects insects, sciencing_icons_plants & mushrooms plants & mushrooms, sciencing_icons_animals animals, sciencing_icons_math math, sciencing_icons_arithmetic arithmetic, sciencing_icons_addition & subtraction addition & subtraction, sciencing_icons_multiplication & division multiplication & division, sciencing_icons_decimals decimals, sciencing_icons_fractions fractions, sciencing_icons_conversions conversions, sciencing_icons_algebra algebra, sciencing_icons_working with units working with units, sciencing_icons_equations & expressions equations & expressions, sciencing_icons_ratios & proportions ratios & proportions, sciencing_icons_inequalities inequalities, sciencing_icons_exponents & logarithms exponents & logarithms, sciencing_icons_factorization factorization, sciencing_icons_functions functions, sciencing_icons_linear equations linear equations, sciencing_icons_graphs graphs, sciencing_icons_quadratics quadratics, sciencing_icons_polynomials polynomials, sciencing_icons_geometry geometry, sciencing_icons_fundamentals-geometry fundamentals, sciencing_icons_cartesian cartesian, sciencing_icons_circles circles, sciencing_icons_solids solids, sciencing_icons_trigonometry trigonometry, sciencing_icons_probability-statistics probability & statistics, sciencing_icons_mean-median-mode mean/median/mode, sciencing_icons_independent-dependent variables independent/dependent variables, sciencing_icons_deviation deviation, sciencing_icons_correlation correlation, sciencing_icons_sampling sampling, sciencing_icons_distributions distributions, sciencing_icons_probability probability, sciencing_icons_calculus calculus, sciencing_icons_differentiation-integration differentiation/integration, sciencing_icons_application application, sciencing_icons_projects projects, sciencing_icons_news news.
- Share Tweet Email Print
- Home ⋅
- Science ⋅
- Biology ⋅
- Ecology: Definition, Types, Importance & Examples

Ecological Research Methods: Observing, Experimenting & Modeling
Ecology is the study of the relationship between organisms and their environment on earth. Several ecological methods are used to study this relationship, including experimenting and modeling.
Manipulative, natural or observational experiments may be used. Modeling helps analyze the collected data.
What Is Ecology?
Ecology , the study of how organisms interact with their environment and each other, draws upon several other disciplines. The environmental science of ecology incorporates biology, chemistry, botany, zoology, mathematics and other fields.
Ecology examines species interactions, population size, ecological niches, food webs, energy flow and environmental factors. In order to do this, ecologists rely on careful methods to collect the most accurate data they can. Once data is collected, ecologists then analyze it for their research.
The information gained from these research methods can then help ecologists find impacts caused by humans or natural factors. This information can then be used to help manage and conserve impacted areas or species.
Observation and Field Work
Every experiment requires observation. Ecologists must observe the environment, the species within it and how those species interact, grow and change. Different research projects require different types of assessments and observations.
Ecologists sometimes use a desk-based assessment , or DBA, to collect and summarize information about specific areas of interest. In this scenario, ecologists are using information already collected from other sources.
Oftentimes, however, ecologists rely on observation and field work . This entails actually going into the habitat of the subject of interest to observe it in its natural state. By doing field surveys, ecologists can track population growth of species, observe community ecology in action and study the impact of any new species or other introduced phenomena in the environment.
Each field site will differ in nature, in shape or in other ways. Ecological methods allow for such differences so that different tools can be used for observations and sampling. It is crucial that sampling be done in a random fashion to combat bias.
Types of Data Obtained
Data obtained from observation and field work can be either qualitative or quantitative. These two classifications of data vary in distinct ways.
Qualitative data : Qualitative data refers to a quality of the subject or conditions . It is therefore a more descriptive form of data. It is not easily measured, and it is collected by observation.
Because qualitative data is descriptive, it might include aspects such as color, shape, whether the sky is cloudy or sunny, or other aspects for how an observation site might look. Qualitative data is not numerical like quantitative data. It is therefore considered less reliable than quantitative data.
Quantitative data: Quantitative data refers to numerical values or quantities . These kinds of data can be measured and are usually in number form. Examples of quantitative data might include pH levels in soil, the number of mice in a field site, sample data, salinity levels and other information in numeric form.
Ecologists use statistics to analyze quantitative data. It is therefore considered a more reliable form of data than qualitative data.
Types of Field Work Surveys
Direct survey: Scientists can directly observe animals and plants in their environment. This is called a direct survey. Even in places as remote as a seafloor, ecologist can study the underwater environment. A direct survey in this case would entail photographing or filming such an environment.
Some sampling methods used to record images of sea life on the seafloor include video sledges, water curtain cameras and Ham-Cams. Ham-Cams are attached to a Hamon Grab, a sample bucket device used to collect samples. This is one effective way to study animal populations.
The Hamon Grab is a method of collecting sediment from the seafloor, and the sediment is taken onto a boat for ecologists to sort through and photograph. These animals will be identified in a laboratory elsewhere.
In addition to a Hamon Grab, undersea collection devices include a beam trawl, which is used to obtain larger sea animals. This entails attaching a net to a steel beam and trawling from the back of a boat. The samples are brought on board the boat and photographed and counted.
Indirect survey: It is not always practical or desirable to observe organisms directly. In this situation, ecological methods entail observing the traces those species leave behind. These could include animal scat, footprints and other indicators of their presence.
Ecological Experiments
The overarching purpose of ecological methods for research is to get high-quality data. In order to do this, experiments must be carefully planned.
Hypothesis : The first step in any experimental design is to come up with a hypothesis or scientific question. Then, researchers can come up with a detailed plan for sampling.
Factors that affect field work experiments include the size and shape of an area that needs to be sampled. Field site sizes range from small to very large, depending on what ecological communities are being studied. Experiments in animal ecology must take into account potential movement and size of animals.
For example, spiders would not require a large field site for study. The same would be true when studying soil chemistry or soil invertebrates. You could use a size of 15 meters by 15 meters.
Herbaceous plants and small mammals might require field sites of up to 30 square meters. Trees and birds might need a couple of hectares. If you are studying large, mobile animals, such as deer or bears, this could mean needing a quite large area of several hectares.
Deciding upon the number of sites is also crucial. Some field studies might require only one site. But if two or more habitats are included in the study, two or more field sites are necessary.
Tools: Tools used for field sites include transects, sampling plots, plotless sampling, the point method, the transect-intercept method and the point-quarter method. The goal is to get unbiased samples of a high-enough quantity that statistical analyses will be sounder. Recording information on field data sheets aids in the data collection.
A well-designed ecological experiment will have a clear statement of purpose or question. Researchers should take extraordinary care to remove bias by providing both replication and randomization. Knowledge of the species being studied as well as the organisms within them is paramount.
Results: Upon completion, collected ecological data should be analyzed with a computer. There are three types of ecological experiments that can be made: manipulative, natural and observational.
Manipulative Experiments
Manipulative experiments are those in which the researcher alters a factor to see how it affects an ecosystem. It is possible to do this in the field or in a laboratory.
These kinds of experiments provide interference in a controlled manner. They work in cases in which field work cannot occur over an entire area, for various reasons.
The downside of manipulative experiments is they are not always representative of what would happen in the natural ecosystem. Additionally, manipulative experiments might not reveal the mechanism behind any patterns observed. It is also not easy to change variables in a manipulative experiment.
Example : If you wanted to learn about lizard predation of spiders, you could alter the number of lizards in enclosures and study how many spiders resulted from this effect.
A larger and current example of a manipulation experiment is the reintroduction of wolves into Yellowstone National Park. This reintroduction allows for ecologists to observe the effect of wolves returning to what was once their normal range.
Already, researchers have learned that an immediate change in the ecosystem occurred once wolves were reintroduced. Elk herd behaviors changed. Increased elk mortality led to a more stable food supply for both wolves and carrion eaters.
Natural Experiments
Natural experiments, as their name implies, are not directed by humans. These are manipulations of an ecosystem caused by nature. For example, in the wake of a natural disaster, climate change or invasive species introduction, the ecosystem itself represents an experiment.
Of course, real-world interactions such as these are not truly experiments. These scenarios do provide ecologists with opportunities to study the effects natural events have on species in an ecosystem.
Example: Ecologists could take a census of animals on an island to study their population density.
The main difference between manipulative and natural experiments from a data perspective is that natural experiments do not have controls. Therefore it is sometimes harder to determine cause and effect.
Nevertheless, there is useful information to be gained from natural experiments. Environmental variables like moisture levels and density of animals can still be used for data purposes. Additionally, natural experiments can occur across large areas or vast stretches of time. This further distinguishes them from manipulative experiments.
Unfortunately, humanity has caused catastrophic natural experiments across the globe. Some examples of these include habitat degradation, climate change, introduction of invasive species and removal of native species.
Observational Experiments
Observational experiments require adequate replications for high-quality data. The “rule of 10” applies here; researchers should collect 10 observations for each category required. Outside influences can still hamper efforts to collect data, such as weather and other disturbances. However, using 10 replicating observations can prove helpful for obtaining statistically significant data.
It is important to perform randomization, preferably prior to performing observational experiments. This can be done with a spreadsheet on a computer. Randomization strengthens data collection because it reduces bias.
Randomization and replication should be used together to be effective. Sites, samples and treatments should all be randomly assigned to avoid confounded results.
Ecological methods rely heavily on statistical and mathematical models. These provide ecologists with a way to predict how an ecosystem will change over time or react to changing conditions in the environment.
Modeling also provides another way to decipher ecological information when field work is not practical. In fact, there are several drawbacks to relying solely on field work.Because of the typically large scale of field work, it is not possible to replicate experiments exactly. Sometimes even the lifespan of organisms is a rate-limiting factor for field work. Other challenges include time, labor and space.
Modeling, therefore, provides a method in which to streamline information in a more efficient manner.
Examples of modeling include equations, simulations, graphs and statistical analyses. Ecologists use modeling for producing helpful maps as well. Modeling allows for calculations of data to fill in gaps from sampling. Without modeling, ecologists would be hampered by the sheer amount of data that needs to be analyzed and communicated. Computer modeling allows for comparatively rapid analysis of data.
A simulation model, for example, enables the description of systems that would otherwise be extremely difficult and too complex for traditional calculus. Modeling allows scientists to study coexistence, population dynamics and many other aspects of ecology. Modeling can help predict patterns for crucial planning purposes, such as for climate change.
Humanity’s impact upon the environment will continue. It therefore becomes ever more crucial for ecologists to use ecological research methods to find ways to mitigate the effects on the environment.
Related Articles
Distinguishing between descriptive & causal studies, advantages and disadvantages of quadrat use, importance of an eia in environmental protection, tools used in hydrology, slovin's formula sampling techniques, what is pps sampling, laboratory observation methods, how does sediment affect ecosystems, how to test linearity in spss, how to determine sample size, internal & external control in experiments, definitions of control, constant, independent and dependent..., topics for experiments in ecology, steps & procedures for conducting scientific research, 10 characteristics of a science experiment, what is the meaning of variables in research, spider adaptations, why should you only test for one variable at a time....
- Wessex Archaeology: Explore the Seafloor: Ecological Research Methods
- EcologyandEvolution.org: How to Design a Field Study
- The University of Vermont: Designing Successful Field Studies
- MyYellowstonePark.com: Wolf Reintroduction Changes Ecosystem in Yellowstone
- Oxford Bibliographies: Simulation Modeling
- University of Ohio: Intro to Ecology and Experiments
- Clever ISM: Overview of Qualitative and Quantitative Data Collection Methods
About the Author
J. Dianne Dotson is a science writer with a degree in zoology/ecology and evolutionary biology. She spent nine years working in laboratory and clinical research. A lifelong writer, Dianne is also a content manager and science fiction and fantasy novelist. Dianne features science as well as writing topics on her website, jdiannedotson.com.
Find Your Next Great Science Fair Project! GO
User Preferences
Content preview.
Arcu felis bibendum ut tristique et egestas quis:
- Ut enim ad minim veniam, quis nostrud exercitation ullamco laboris
- Duis aute irure dolor in reprehenderit in voluptate
- Excepteur sint occaecat cupidatat non proident
Keyboard Shortcuts
7.2 - ecological studies, rationale and ecological variables section .
An ecological study is an observational study in which at least one variable is measured at the group level. An ecological study is especially appropriate for the initial investigation of causal hypotheses. So...why conduct an ecological study? Several reasons support using an ecological study design.
- The hypothesis is relatively new
- Adequate measurement of individual-level variables is not possible
- Adequate design of an individual-level study is not possible (i.e., not ethical)
- We are interested in the effect of ecological variables, for which there is no correlation at the individual level
- We have limited funds or limited time to do the study
Three types of ecological variables:
Advantages & disadvantages of ecological studies.
- Can be done quickly and inexpensively bc rely on pre-existing data
- Analysis and presentation are relatively simple
- Can achieve a wider range of exposure levels than can be expected from an individual-level study
- Help explain population-level associations
Disadvantages
- Ecological fallacy - the possibility of making incorrect conclusions about individual-level associations when only using group-level data
- Lack of information on important variables
Analysis of Ecologic Studies
Analytic models in ecologic studies are of different forms:
Examples in Research Section
Effectiveness of needle-exchange programmes for prevention of HIV infection
Egg consumption and mortality from colon and rectal cancers: an ecological study
International comparisons of prostate cancer mortality rates with dietary practices and sunlight levels
Click through the PLOS taxonomy to find articles in your field.
For more information about PLOS Subject Areas, click here .
Loading metrics
Open Access
Peer-reviewed
Research Article
Trends in Ecological Research during the Last Three Decades – A Systematic Review
Affiliation Faculty of Civil and Environmental Engineering, Technion – Israel Institute of Technology, Haifa, Israel
* E-mail: [email protected]
Affiliation Department of Plant Sciences, University of Cambridge, Cambridge, United Kingdom
Affiliation Department of Biology and Environment, University of Haifa at Oranim, Kiryat Tivon, Israel
Affiliation Institute of Evolution, University of Haifa, Haifa, Israel
- Yohay Carmel,
- Rafi Kent,
- Avi Bar-Massada,
- Lior Blank,
- Jonathan Liberzon,
- Oded Nezer,
- Gill Sapir,
- Roy Federman
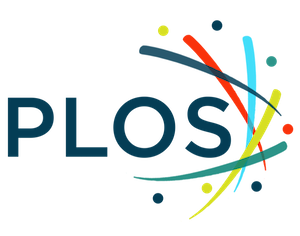
- Published: April 24, 2013
- https://doi.org/10.1371/journal.pone.0059813
- Reader Comments
It is thought that the science of ecology has experienced conceptual shifts in recent decades, chiefly from viewing nature as static and balanced to a conception of constantly changing, unpredictable, complex ecosystems. Here, we ask if these changes are reflected in actual ecological research over the last 30 years. We surveyed 750 articles from the entire pool of ecological literature and 750 articles from eight leading journals. Each article was characterized according to its type, ecological domain, and applicability, and major topics. We found that, in contrast to its common image, ecology is still mostly a study of single species (70% of the studies); while ecosystem and community studies together comprise only a quarter of ecological research. Ecological science is somewhat conservative in its topics of research (about a third of all topics changed significantly through time), as well as in its basic methodologies and approaches. However, the growing proportion of problem-solving studies (from 9% in the 1980s to 20% in the 2000 s) may represent a major transition in ecological science in the long run.
Citation: Carmel Y, Kent R, Bar-Massada A, Blank L, Liberzon J, Nezer O, et al. (2013) Trends in Ecological Research during the Last Three Decades – A Systematic Review. PLoS ONE 8(4): e59813. https://doi.org/10.1371/journal.pone.0059813
Editor: Luís A. Nunes Amaral, Northwestern University, United States of America
Received: September 16, 2012; Accepted: February 19, 2013; Published: April 24, 2013
Copyright: © 2013 Carmel et al. This is an open-access article distributed under the terms of the Creative Commons Attribution License, which permits unrestricted use, distribution, and reproduction in any medium, provided the original author and source are credited.
Funding: This study was funded by the Israel Science Foundation (grant number 486-2010). The funders had no role in study design, data collection and analysis, decision to publish, or preparation of the manuscript.
Competing interests: The authors have declared that no competing interests exist.
Introduction
Ecologists often describe ecological science as dynamic. ‘Ecology is a science in transition’ [1] . This transition is characterized by several significant shifts in emphasis and perspective [2] . During most of the 20 th century, the majority of ecologists conceptualized ecological systems as balanced and stable, typically at equilibrium, or as returning to such equilibrium deterministically following rare disturbances [3] . In recent decades, there has been a shift towards an understanding of ecological systems as nonlinear, constantly changing, and unpredictable in time and space [4] , [5] . The concept of equilibrium was replaced by other concepts, for example, the concept of non-equilibrium change, in which the system is often described as rotating between alternative states [6] .
Ecologists are split on the question of whether the changes in ecological science represent a Kuhnian ‘paradigm shift’ [5] , [7] , [8] , [9] , or, alternatively, a gradual accumulation of modifications, better characterized as ‘evolution’ rather than ‘revolution’ [2] , [10] . In contrast, other ecologists maintained that progress in ecology is lacking [11] or limited [12] .
Here, we ask if the topics and methodologies of ecological research as reflected in the literature of the last 30 years provide evidence to support notions of dramatic shifts, or of gradual change. We characterize various aspects of ecological research, using an extensive survey of ecological literature. In particular, we ask three questions regarding general aspects of ecology, and look for possible changes in these aspects over the last 30 years:
- Domains of ecological research : What proportion of research is devoted to the various domains in ecology (population, species, community, and ecosystem)? What are the major topics of ecological study? Has there been a change in the frequency of investigation of any of these topics and, if so, which ones?
- Types of research : Is ecology an experimental science, or a science of observation and measurement? How often are models used in ecological research? To what degree do ecologists use meta-analysis of data from previous studies (vs. collecting new data in each research)?
- Basic science or problem-solving oriented discipline : Is ecology becoming a problem-solving science? In other words, how often does ecology relate to actual, specific environmental problems, in an attempt to provide solutions (or at least new insights on how to make progress towards solutions)?
Preliminary expectations
A. domains of ecological research..
The concepts of ecosystem and community have become increasingly dominant in ecological thinking. In a survey conducted among members of the British Ecological Society, ecosystem was identified as the single most important concept in ecology [13] . More recently, the Ecological Visions Committee of the Ecological Society of America issued a report that listed eight critical environmental issues for prioritizing ecological research [14] . Only two of those topics related to populations and species, while five topics were clearly within the domains of ecosystems and communities . We expected an increase in research conducted at the ecosystem level, and at the community level, accompanied by a proportional decrease in studies of single species. We also expected specific topics to become more frequent subjects of ecological study (such as biodiversity, climate change, biogeochemistry, and scale).
B. Types of research.
Observations and experiments are known to be the two dominant tools of ecological research . In this research, we expected to identify an increase in the frequency of models, for two reasons: (1) the ecosystem has increasingly been described as ‘complex’, and models are often the only tools available for the study of complex systems, and (2) due to the substantial increase in the availability of modelling tools during the last three decades. We also expected an increase in the proportion of meta-analysis studies, for two major reasons: (1) a growing awareness of the incapacity of single studies of specific systems, conducted under narrow ranges of conditions, to provide insights on broader ecological issues [15] , and (2) the increased access to information and data in the age of the Internet.
C. Is ecology a problem-solving science?
In the past, ecologists have been reluctant to engage in applied research [16] . Applied science was considered inferior to basic, ‘pure’ science [17] . Some applied ecological issues, such as conservation, are emotionally charged [2] , and perceived by some ecologists as ‘advocacy’ [18] . More recently, ecologists have become increasingly concerned about the implications of their work to society's problems [15] , [17] , while environmental agencies have expressed an increased demand for ecological solutions to environmental problems [19] . For these reasons, we expected to find an increase in the proportion of applied studies over the last three decades.
In order to attempt to answer these questions, a quantitative survey of ecological research is required. Surprisingly, few attempts have been made to systematically quantify trends in ecological research. Typically, these studies have used an automated count of words in titles and abstracts to assess trends in ecology [20] , [21] , [22] , [23] . Shorrocks [24] used an alternative method to survey trends in ‘the Journal of animal ecology’ –he actually sampled 13 volumes of the journal between 1932 and 1992. Here, we followed that method: we inspected a large sample of the ecological literature, classifying it according to its content. This process is time-consuming, but the resulting analysis is probably more reliable than an automated word count.
Two surveys
One major consideration was our choice of target population within the ecological literature. Two plausible alternatives existed: we could either sample the entire pool of ecological research, or sample only leading journals. There are pros and cons to each choice. Including the entire range of ecological literature may introduce research of varying quality into the analysis. On the other hand, niche journals (the vanguard of novel research) may serve as early indicators of transitions and trends. We therefore decided to conduct two parallel surveys, using identical methods. In survey 1, we included all 136 journals that concern ecology, while in survey 2 we sampled eight ‘core journals’ that were published throughout the entire study period. A brief description of the data collection approach can be found in the Prisma 2009 flow diagram and checklist.
Journal selection
For survey 1, we selected all relevant journals that appeared during at least parts of the study period 1981–2010. This pool consisted of 136 journals. From the entire collection of articles published in these journals during this period, we limited the selection to research articles in English, and received a total of 110,965 articles (see Appendix S1 for a full list of journals sampled for this survey).
For survey 2, we selected eight prominent journals, using the following criteria: (a) high-impact factor (among the top 30 ecological journals, using ISI Web of Science Impact Factor), (b) generality (cover the entire scope of ecological research), and (c) consistency (were published throughout the study period). Not a single journal satisfied all three criteria. We therefore selected eight journals belonging to three major ecological societies that issue their own journals; thus, each group, as such, satisfies all three criteria. The eight journals were those issued by the Ecological Society of America ( Ecological Applications , Ecological Monographs , and Ecology ); the British Ecological Society (Journal of Ecology, Journal of Animal Ecology, and Journal of Applied Ecology), and the Nordic Ecological Society ( Oikos and Ecography ). Ecological Applications , first published in 1991, was an offshoot of Ecology, and Ecography , first published in 1991, was an offshoot of Oikos ; we assumed that the range of topics covered by each of the pairs was similar to that of the parent journal prior to the split. The pool of all research articles published in these journals in the period 1981–2010 consisted of 22,788 articles).
For each of the two surveys, we used a random selection scheme to select 25 articles from each year, totalling 750 articles in each survey. The classification (domain, topics, research type, applied or basic science) was performed by the authors of the current study, based on the articles. In many cases, reading the abstract provided sufficient information for classifying the article. In order to ensure a high degree of consistency between the classifiers, we carried out a pilot exercise, in which the degree of agreement between the classifiers was assessed prior to the research study. A set of 29 articles was classified independently by all classifiers. Classifications were then discussed until consensus was reached for each classification. For each topic and for each classifier, the level of agreement between initial classification and final ‘consensus’ was recorded.
Article characterization
A. ecological domains..
We predefined 20 topics that describe major research fields in ecology, and grouped these 20 topics into five broad ecological domains: (1) Single Species (demography, physiology, distribution, behaviour, evolution, genetics); (2) Species Interactions (grazing, predation, mutualism, parasitism, competition); (3) Community (biodiversity, community structure); (4) Ecosystem (food web, climate change, vegetation dynamics, biomass and productivity, biogeochemistry); and (5) Other topics (scale, statistics). We limited topic-based characterization to three topics per article.
B. Type of research.
We classified the type of ecological research according to four general categories: experiment, observation, model, and data analysis. An article was classified as ‘experiment’ if an actual experiment was conducted in the laboratory, or if a field study included some sort of treatment or manipulation of the natural environment. Where research included both observation and field experiment, the article was labelled ‘experiment’. ‘Observation’ was a study where the major activity was any sort of measurement of ecological phenomena. An article was labelled ‘model’ if its sole activity or the major endeavor was to construct a model. In cases where a model was only a minor part of the research, the article was labelled ‘experiment’ or ‘observation’. Articles that did not present any new data, but used data collected in previous studies, often conducting meta-analysis, were labelled ‘data analysis’. Articles that did not include any of the above types of research, but discussed ecological issues qualitatively were omitted from the survey (and a replacement was added).
C. Problem-solving.
Our goal here was to determine the degree to which ecology is oriented towards problem-solving. We assigned the category of ‘application’ to all articles that either searched for solutions to problems associated with anthropogenic activities, or proposed tools for practical problems (such as practices for conservation, global change mitigation etc.).
Statistical analyses
The number of articles assigned to each ecological topic, label, and variable was recorded for each survey. The differences between surveys in terms of the frequency of each term were analyzed using Chi square test. To evaluate change in the frequency of these variables over time, we used logistic regression [25] , with publication year as a continuous variable and survey type as a fixed variable. In order to account for multiple comparisons, we applied the Bonferroni correction. Fifty comparisons (25 comparisons in each survey) yielded a threshold of p<0.001 . The Bonferroni correction becomes very conservative when the number of comparisons becomes large, as it controls the probability of false positives only, at the cost of increasing the probability of false negatives [26] . We therefore report the results using Bonferroni correction, as well as for less conservative thresholds.
Classification consistency
Classifiers' results were in good agreement with the consensus of the test articles, with an overall average agreement rate of 90%. The average accuracy of parameter classification was high in all cases, ranging from 86% for ‘topics’ and ‘problem solving’, to 93% and 94% for ‘research type’ and ‘domain’, respectively. In what follows, wherever we report two figures, the first figure refers to the ‘all journals’ survey, and the second figure refers to the ‘core journals’ survey.
Domains of ecological research
(1) Single Species was the most frequent domain of study in this survey of ecological research, with 71% (66%) of all the studies involving topics within this domain ( Table 1 ). In both surveys, the four most common topics related to this domain: demography, physiology, behaviour and distribution. Taken together, these topics appeared in 64% (63%) of all articles. Only 4% (3%) of all articles studied evolution. (2) In the Species Interactions domain, predation and competition were the frequent terms, recorded in 5–9% of the articles, while mutualism and parasitism were recorded in 2–4% of the articles. (3) Community-related topics (biodiversity and community structure) appeared in 17% of the studies in both surveys, and (4) Ecosystem-related topics appeared in nearly a quarter of the articles. Among ecosystem topics, biogeochemistry accounted for 11% (8%) of ecological research and 2% of the research concerned climate change studies ( Table 1 ).
- PPT PowerPoint slide
- PNG larger image
- TIFF original image
https://doi.org/10.1371/journal.pone.0059813.t001
The frequency of community studies increased significantly (nearly significantly in the ‘core journals’) during the studied period, while the other three domains remained quite constant over time ( Table 2 , Figure 1 ).
White bars denote ‘all journals’ and gray bars denote ‘core journals’. Temporal trend was significant for community studies only (a logistic model, see Table 2 ).
https://doi.org/10.1371/journal.pone.0059813.g001
https://doi.org/10.1371/journal.pone.0059813.t002
There were significant changes in the frequency of several topics over time. The frequency of two topics climate change and biodiversity, increased significantly with time in both surveys ( Table 2 , Figure 2 ). The frequency of three additional topics changed significantly in the ‘all journals’ survey only: physiology and behaviour decreased, while genetics increased. An increase in the frequency of scale was the single significant change that appeared in the ‘core journals’ only. Additionally, five topics revealed a nearly significant frequency change through time in that survey (p<0.05): demography , grazing , and vegetation dynamics decreased, while evolution and parasitism increased in frequency with time ( Table 2 ).
White bars denote ‘all journals’ and gray bars denote ‘core journals’. *** Temporal trend was significant (a logistic model, see Table 2 ), p<0.001. ** p<0.01. * p<0.05.
https://doi.org/10.1371/journal.pone.0059813.g002
Differences between the two surveys
The results of both surveys were quite similar for 14 of the 20 topics, while significant differences between the two surveys were found for six topics: physiology , behaviour and genetics were much more frequent in the ‘all journals’ survey, while demography , grazing , and vegetation dynamics were much more frequent in the ‘core journals’ survey ( Table 1 ). Most domains appeared at similar frequencies in the two surveys, except Species Interactions, which was nearly twice as frequent in the ‘core journals’ survey compared to its frequency in the ‘all journals’ survey ( Table 1 ).
Type of research
Observations constitutes the major type of ecological research (59%, 45%), followed by experiments (28%, 36%), while models (12%, 12%) and data-analysis (9%, 6%) were less frequent ( Table 3 ).
https://doi.org/10.1371/journal.pone.0059813.t003
The proportion of data-analysis studies increased significantly with time in the ‘all journals’ survey. The use of models as a primary research tool slightly decreased in ‘all journals’ and slightly increased (nearly significant) in the ‘core journals’ survey ( Table 4 ).
https://doi.org/10.1371/journal.pone.0059813.t004
Observation studies were significantly more frequent in the ‘all journals’ survey, while experiments were somewhat more frequent in the ‘core journals’ survey.
Is ecology a problem-solving science?
Overall, 17% (15%) of the articles were labelled ‘problem solving’ ( Table 3 ). In both surveys, their proportion increased significantly over time, from 9% (7%) in the 1980s to 21% (21%) in the 2000 s ( Table 4 , Figure 2 ).
Few systematic surveys of ecological literature have been conducted to date, and most have been restricted to a single theme or a narrow branch of ecological science [20] , [21] , [22] , [23] . For example, [22] evaluated relations between the size of the organism and its relative representation in ecological research. Swihart [12] quantified the rates of appearance of new ecological terms and disappearance of old terms. Shorrocks [24] was perhaps the only investigator to quantify various trends in ecological science, using articles published in The Journal of Animal Ecology between 1932 and 1992. To the best of our knowledge, the present study is the first attempt to systematically survey the entire breadth of ecological literature, in order to quantify various characteristics of the science of ecology, as well as their temporal trends. The results suggest that ecology may be substantially less dynamic than is generally acknowledged.
Ecology is mostly a study of single species. Most of the ecological research focused on the demography, physiology and distribution of single species. The proportion of single-species studies has slightly decreased in the past three decades, but still consists of more than 60% of the studies. In comparison, community and ecosystem studies represented a minor fraction of ecological research. This surprising finding seems at odds with the strong emphasis on the community and the ecosystem as major concepts in ecology [27] , [28] . Also surprising was the scarcity of a few topics which are thought to be central in ecology. Two notable examples are evolution, and food-web, each of which appeared as a research topic in 2–4% of the articles. Most of the increase in community studies occurred in the 2000s, probably reflecting the renewed interest in this field, after the neutral theory challenged the prevalence of the niche concept.
The analysis of changes in the frequency of research topics over time provided inconclusive results. Only two topics, climate change and biodiversity, showed a significant change in both surveys. The increase in both topics probably relates to the fact that both were non-issues at the beginning of the period under study. Four other topics changed significantly, and seven other topics changed nearly significantly, in only one of the surveys. Overall, there does not seem to be a drastic transformation in the relative importance of domains and topics in the field of ecology, but the apparent change in topics and research types signifies that ecological science is not entirely stagnant.
The frequency of more than half of the topics and domains was very similar in both surveys, but nearly a third of the topics differed significantly between the surveys. Interestingly, the topics that were significantly more frequent in the ‘all journals’ survey related to the basic and static aspects of a species (genetics and physiology), and the ecosystem (biomass and productivity). In contrast, the topics that were significantly more frequent in the ‘core journals’ related to dynamic processes (demography, vegetation dynamics, and grazing).
Observation and experiment were by far the predominant tools of ecological study, together accounting for 80% of the research; these proportions did not change over time. Interestingly, modelling (∼12% of all studies), is no more common today than it was thirty years ago, despite a drastic increase in the availability of modelling tools during this period. Data-analysis became a more common research tool. Many of the studies in this category were, in fact, meta-analyses (analyses of data from several sources). The major increase in data-analysis studies was in the mid-90s, suggesting that the increased availability of information in the age of the Internet had an important role in this trend.
Comparing the two surveys in terms of type of research revealed a fundamental difference: the ratio of experiments to observations in the ‘all journals’ survey was 1:2, while in the ‘core journals’ survey it was 7:9. The prevalent consensus that ecology has changed during the 20 th century, from an observational to an experimental science, may be somewhat overstated; nevertheless, such a change appeared more prominently in the ‘core journals’ survey.
Ecological research is mostly a basic science, with only a small proportion of ‘problem solving’ studies. Yet, in both surveys we found a significant and consistent increase in the number of ‘problem solving’ articles published during the survey period. If this trend continues in future decades, it may prove to be a major shift in the orientation of ecology.
Is ecology a dynamic science?
Prominent ecologists have claimed that ecology has undergone transitions [29] , and even paradigm shifts [5] in recent decades, and is now a mature and competent science [30] . Our survey reveals that these claims perhaps overstate the case. The science of ecology appears to be changing slowly, in the sense that major research subjects and principal methodologies have not changed dramatically for at least 30 years. In particular, the popular image of ecology as a science in transition [7] , dealing chiefly with ecosystems and communities [1] seems at odds with the major proportion of single species studies reported here.
A contrasting view, put forward by O'Connor [11] , claimed that ecology lags after other life sciences, and makes very little progress. O'Conner's study ignited a debate, wherein various arguments were employed to disprove this claim [31] , [23] , or put it in a balanced perspective [12] . This debate is still ongoing, and is probably driven by emotions no less than by objective evaluations. The current study does not substantiate O'Connor's claim, and it was not meant to evaluate progress. However, it is safe to assume that a major advance in ecology would be accompanied by a major change in the frequency of domains, topics, and types of research; yet, as shown here, these have changed only moderately in the course of three decades.
A major aspect of progress in science is the rate at which basic questions in ecology are being answered [12] , which we have not evaluated, and is very difficult to evaluate quantitatively. Also, we could not detect conceptual shifts, such as network thinking, that do not connect to particular terms or topics. Swihart et al. [12] provide an interesting attempt to quantify progress based on ‘birth rate’ and ‘death rate’ of ecological terms, and claim to show viable progress in ecology. In contrast, the list of 100 fundamental questions in ecology [32] reports profound knowledge gaps regarding the central mechanisms driving ecosystems, communities, and even population dynamics.
Our approach could not, and was not meant to detect changes in particular methods and technologies applied within each research domain or topic. The availability of advanced molecular and genetic tools and the increase in computing power have allowed analyses to become more complex and sophisticated. However, the use of these new technologies and processing power does not imply enhanced knowledge or understanding. Also, such surveys may not detect conceptual shifts, such as network thinking, which do not connect to particular terms or topics.
Perhaps the single and most important change in the study of ecology is the growing proportion of ecological research directed towards problem solving. This trend by itself, if continued, may represent a major transition in ecology in the long run.
Our results may be disturbing to some researchers, insofar as they portray an ecological discipline which is considerably less dynamic than ecologists would like to believe. The value of this research is precisely in reviving the debate and presenting an opportunity for self-assessment to those who strive to advance the discipline, all of which can serve to stimulate the investigation of new and groundbreaking tools, paradigms and perspectives. Only through meta-scale monitoring of the scope of research can we understand, and hope to influence, the trajectory of ecological research in the years to come.
Supporting Information
Appendix s1..
A full list of journals sampled for survey 1.
https://doi.org/10.1371/journal.pone.0059813.s001
Flow Diagram S1.
https://doi.org/10.1371/journal.pone.0059813.s002
Checklist S1.
https://doi.org/10.1371/journal.pone.0059813.s003
Acknowledgments
Curtis Flather, Mark Burgman, Leon Blaustein, Yaacov Garb, Yaron Ziv and Daniel Statman have provided valuable comments on a draft of this manuscript.
Author Contributions
Conceived and designed the experiments: YC AB LB RF. Performed the experiments: YC RK AB LB JL ON RF GS. Analyzed the data: RF YC GS. Wrote the paper: YC RK AB LB RF JL ON.
- View Article
- Google Scholar
- 2. Wallington TJ, Hobbs RJ, Moore SA (2005) Implications of current ecological thinking for biodiversity conservation: A review of the salient issues. Ecology and Society 10.
- 4. Ostfeld RS, Pickett STA, Shachak M, Likens GE (1997) The ecological basis of conservation: heterogeneity, ecosystems and biodiversity. New York: Chapman & Hall.
- 5. Botkin DB (1990) Discordant harmonies:a new ecology for the 21st century. New York: Oxford University Press.
- 9. Pickett STA, Parker VT, Fielder P (1992) The new paradigm in ecology: implications for biology above the species level. In: Fielder P, Jain S, editors. Conservation biology: practice of nature conservation. New York, NY: Chapman and Hall. 65–88.
- 14. Palmer M, Bernhardt E, Chornesky E, Collins S, Dobson A, et al.. (2004) Ecological science and sustainability for a crowded planet: 21st century vision and action plan. The Ecological Society of America.
- 16. Hobbs RJ (1998) Managing ecological systems and processes. In: Peterson D, Parker VT, editors. Scale Issues in Ecology. New York: Columbia University Press. pp.459–484.
- 24. Shorrocks B (1993) Trends in the Journal of Animal Ecology: 1932–92. Journal of Animal Ecology: 599–605.
- 25. Sokal RR, Rohlf FJ (1995) Biometry. New York: W.H. Freeman & Company. 887 p.
- 26. Abdi H (2007) Bonferroni and‥ id T: ¡k corrections for multiple comparisons.λ In NJ Salkind (ed.). Encyclopedia of Measurement and Statistics. Thousand Oaks, CA: Sage.
- 28. Lawton JH (2000) Community ecology in a changing world. Oldendorf/Luhe: Ecology Institute.
- 29. Pickett STA, White PS (1985) Patch dynamics: A synthesis. In: Pickett STA, White RS, editors. The ecology of natural disturbance and patch dynamics. London: Academic Press. pp.371–384.

PH717 Module 1B - Descriptive Tools
Descriptive epidemiology & descriptive statistics.
- Page:
- 1
- | 2
- | 3
- | 4
- | 5
- | 6
- | 7
- | 8
- | 9

Ecological Studies (Correlational Studies)
Test yourself.

Ecologic studies assesses the overall frequency of disease in a series of populations and looks for a correlation with the average exposure in the populations. These studies are unique in that the analysis is not based on data on individuals. Instead, the data points are the average levels of exposure and the overall frequency of disease in a series of populations. Therefore, the unit of observation is not a person ; rather, it is an entire population or group.
In the study below investigators used commerce data to compute the overall consumption of meat by various nations. They then calculated the average (per capita) meat consumption per person by dividing total national meat consumption by the number of people in a given country. There is a clear linear trend; countries with the lowest meat consumption have the lowest rates of colon cancer, and the colon cancer rate among these countries progressively increases as meat consumption increases.

Note that in reality, people's meat consumption probably varied widely within each nation, and the exposure that was calculated was an average that assumes that everyone ate the average amount of meat. This average exposure was then correlated with the overall disease frequency in each country. The example here suggests that the frequency of colon cancer increases as meat consumption increases. The characteristic of ecological studies that is most striking is that there is no information about individual people. If the data were summarized in a spread sheet, you would not see data on individual people; you would see records with data on average exposure in multiple groups .
|
an effect in an individual, the exposure and effect must occur in the same person, but , so one does not know if the diseased people were exposed. Consider the following example: Since you don't have any information about the risk factor status or the outcome status of individual people, you can't directly link the risk factor to the disease, i.e., it is not clear that the people who ate the most meat were the ones who got colon cancer. This is sometimes referred to as "ecological bias" or
|
An ecological study correlated per capita alcohol consumption to death rates from coronary heart disease (CHD) in different countries, and it appeared that there was a fairly striking negative correlation as shown in the graph below.

However, a cohort study with data on alcohol consumption in individual subjects showed that there was a J-shaped relationship. People who drank modestly had a lower mortality rates than those who did not drink at all, but among higher levels of individual consumption there was a striking linear increase in mortality, as shown in the graph below.

Source: Adapted from AR Dyer et al. Alcohol consumption and 17-year mortality in the Chicago Western Electric Company Study. Prev. Med . 1980; 9(1):78-90.
The real question was whether individuals who drank heavily had higher or lower mortality rates than those who drank modestly or not all, but the ecologic study led to an incorrect conclusion because it was based on aggregate data. In reality, most people drink modestly, but mortality rates are much greater in the small number of people who drink very heavily. The misleading conclusion from the ecologic study is an example of the ecologic fallacy.
Is the following statement true or false?
For an exposure to cause a health outcome the exposure must precede the outcome in a given person. This is what is observed in ecologic studies.
To see an extraordinary example of an ecologic study, play the video below created by Hans Rosling. This is a magnificent example that examines the correlation between income and life expectancy in the countries of the world over time. It is also a terrific example of a creative, engaging, and powerful way to display a vast quantity of data.

return to top | previous page | next page
Content ©2020. All Rights Reserved. Date last modified: September 10, 2020. Wayne W. LaMorte, MD, PhD, MPH
- Chapter 6. Ecological studies
Geographical comparisons
Time trends, occupation and social class.
- Chapter 1. What is epidemiology?
- Chapter 2. Quantifying disease in populations
- Chapter 3. Comparing disease rates
- Chapter 4. Measurement error and bias
- Chapter 5. Planning and conducting a survey
- Chapter 7. Longitudinal studies
- Chapter 8. Case-control and cross sectional studies
- Chapter 9. Experimental studies
- Chapter 10. Screening
- Chapter 11. Outbreaks of disease
- Chapter 12. Reading epidemiological reports
- Chapter 13. Further reading
Follow us on
Content links.
- Collections
- Health in South Asia
- Women’s, children’s & adolescents’ health
- News and views
- BMJ Opinion
- Rapid responses
- Editorial staff
- BMJ in the USA
- BMJ in Latin America
- BMJ in South Asia
- Submit your paper
- BMA members
- Subscribers
- Advertisers and sponsors

Explore BMJ
- Our company
- BMJ Careers
- BMJ Learning
- BMJ Masterclasses
- BMJ Journals
- BMJ Student
- Academic edition of The BMJ
- BMJ Best Practice
- The BMJ Awards
- Email alerts
- Activate subscription
Information
Thank you for visiting nature.com. You are using a browser version with limited support for CSS. To obtain the best experience, we recommend you use a more up to date browser (or turn off compatibility mode in Internet Explorer). In the meantime, to ensure continued support, we are displaying the site without styles and JavaScript.
- View all journals
- Explore content
- About the journal
- Publish with us
- Sign up for alerts
- Open access
- Published: 06 September 2024
An ecological approach to understanding transitions and tensions in complex learning contexts
- Luke McCrone ORCID: orcid.org/0000-0003-4133-1853 1 &
- Martyn Kingsbury 1
npj Science of Learning volume 9 , Article number: 54 ( 2024 ) Cite this article
51 Accesses
Metrics details
- Human behaviour
The move away from transmission-based lecturing toward a more student-centred active learning approach is well evidenced in STEM higher education. However, the examination of active learning has generally remained confined to formal timetabled contexts, with assumptions made that students independently manage the transition between timetabled and non-timetabled learning. This paper introduces research findings from a mixed methods study that used an ecological approach when investigating student transitions between a formal lecture theatre and adjacent informal breakout space in a UK STEM university. Using quantitative occupancy monitoring data to analyse usage patterns of both spaces, in combination with qualitative ethnographic observations and field interviews, permitted a purposeful exploration of student engagement with transitions within and between the two learning spaces. The ecological approach aided the discovery of spatial, pedagogic and agentic transitions and tensions, which subsequently informed strategic modification of space across the institution to facilitate the adoption of active learning pedagogy.
Similar content being viewed by others
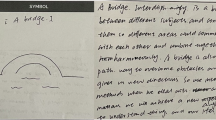
Creating Edinburgh: diffracting interdisciplinary learning and teaching in the contemporary city
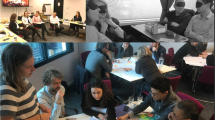
The mixed classroom: a pedagogical experiment with students and policymakers
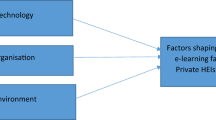
Factors influencing the use of e-learning facilities by students in a private Higher Education Institution (HEI) in a developing economy
Introduction.
There is increasingly widespread recognition in UK higher education that traditional transmission lecturing is less effective than more student-centred active learning, particularly in science, technology, engineering, and mathematics (STEM) fields 1 , 2 . Active learning aligns with the social constructivist perspective 3 , where instructional activities require learners to actively construct knowledge and integrate it with existing knowledge and experiences 4 . A separate body of literature has investigated and evidenced the relationship between this learning activity and the role of physical space 5 . Traditional learning spaces like lecture theatres that are designed for transmission-based pedagogies present challenges for enabling more active pedagogies 6 . The development of active learning classrooms, for example, signals a gradual shift away from the spatial and pedagogic assumptions underpinning these traditional approaches, which have prevailed since the time they were used for ancient oral traditions 7 . However, much of the literature has focussed on active learning within formal timetabled contexts 2 , with assumptions made that students independently manage transitions across timetabled and non-timetabled learning 8 . Turning our attention to informal learning spaces 9 , particularly those spaces adjacent to formal classrooms like corridors 10 , can help us to develop a more holistic understanding of active learning and the challenges of transitioning in both space and pedagogic intent.
The institution researched in this paper has grappled with transforming its curriculum, pedagogy and built estate to achieve its strategic objectives linked to active learning, technology enhancement, and equality, diversity and inclusion. To gain insight into the impact of these strategic changes, the present research explored how students physically transition between timetabled and non-timetabled learning spaces 8 . However, the underlying institutional tension between promoting active pedagogies and teaching those pedagogies within traditional educational infrastructure, whilst not impossible, presented challenging complexity. This tension is particularly pertinent as pedagogies and spaces diversify with the emergence of hybrid, apprenticeship-based and lifelong learning and the evolution of spaces that blur boundaries such as makerspaces 11 . With ‘misunderstanding’ being considered one of three drivers of unsustainable development 12 , there is first a need to discard less appropriate models of how complex, dynamic systems such as universities truly work. Several scholars, such as Ronald Barnett 13 and Ian Kinchin 14 , have consequently challenged the higher education sector to think more ecologically. By drawing from ‘ecology’, a biological term used to inspect the complexity of the interrelationship between organisms and their environment 16 , they argue that we stand a better chance of addressing important issues relating to sustainability, widening participation and lifelong learning while equipping students for rapidly changing future needs and challenges 12 .
Ecological principles have been applied to human social and mental activity since the 1970s 17 and can be similarly drawn upon in this context: interconnectedness, systems thinking, resilience, continuous learning, sustainability, and biodiversity of people and ideas 15 . Ecological systems theory, for example, has broadened our understanding of human development, positing that individuals shape their own learning while simultaneously influencing other people and activities in both their immediate and more remotely connected environments 18 , 19 . This thinking has since extended to ‘ecological leadership’ to perceive leaders as members embedded and distributed within the ecosystem who can become aware of the institution’s ‘natural history’ 14 . This contextual history is best uncovered using ethnography to retrieve a diversity of voices from different settings and to observe the activities and beliefs of those living in those settings 20 . The notion of an ‘ecological university’ therefore embraces the imagination and creativity of individual learners who transform ideas, relationships, materials, and themselves while supporting actors in gaining an understanding of their context, each other, and the epistemic environments in which they learn 21 . Common-pool resource theory posits that the collective management of tangible and intangible resources, such as physical and social spaces, can maximise sustainable output and foster a sense of ‘place’ in universities 22 . Providing opportunities and conditions in which students can cultivate a shared sense of ownership and agency in spaces therefore seems to be important 23 and can be supported via participatory design-based approaches 10 and other initiatives that distribute leadership 24 .
In setting out to study physical transitions between a lecture theatre and adjacent informal space, we soon realised from our initial data that students were navigating several transitions. Therefore, in this paper, ‘transition’ refers both to students moving between these physical spaces, as well as to changes in pedagogic intent, such as transitioning from teacher-centred didactic delivery to student-centred group-based learning. ‘Tensions’ represent the conflicting pressures that often drive or result from these changes. We therefore use the terms transition and tension in this paper to describe multiple phenomena. By understanding how students are engaging with these transitions and tensions between formal and adjacent spaces, this paper seeks to make a unique contribution to our understanding of active learning and pedagogic space. We argue that through the use of mixed methods 25 and an ecological approach, the institution became more aware of these transitions and tensions within the context of its natural history 14 . The ecological approach has assisted in understanding the complex interaction between learning space, pedagogy and agency, as well as the conditions under which students can actively learn. This awareness has informed subsequent modifications to institutional spaces as part of a more sustainable, inclusive and productive learning ecology.
Spatial transitions and tensions
Lecture theatre and adjacent breakout space occupancy data were analysed in the context of the timetable to better understand the relative usage patterns before and after timetabled activity; the term ‘spatial’ is hence used here to describe the intersection between the physical spaces and the timetable. We defined two types of frequently recurring timetable configurations, type 1 and type 2 (see Fig. 1 ), to study transitions between the two spaces in different contexts. The nature of student engagement with and pedagogic potential of these transition periods in each timetable configuration was subsequently further investigated using a mixed methods approach. This approach generated data that informed the conceptualisation of these transition periods as on-ramp (space and time just before the timetabled lecture) and off-ramp (space and time just after the timetabled lecture) transitions, labelled in Fig. 1 .

Definition of type 1 and type 2 timetable configurations that were repeatable and analysable for the lecture theatre with on-ramp and off-ramp transitions labelled.
The group of students ‘meaningfully’ engaging in on-ramp and/or off-ramp transitions was defined as the percentage of the incoming or outgoing lecture cohort who remained in the breakout space for longer than 5 min. These transitions are annotated as opposing arrows in Fig. 2 which displays a type 2 timetable occupancy profile to mark periods in which student cohorts seemed to enter or exit the lecture theatre and gather in the adjacent breakout space. The architectural divide between the two spaces resulted in predictable inflows and outflows of users that were able to be visualised using occupancy plots (like in Fig. 2 ).

Lecture theatre and breakout space occupancy plot labelled with type 2 timetable and occupancy changes as cohorts transition into (on-ramp) and out of (off-ramp) the lecture theatre.
Although our interest was primarily in students associated with the lecture cohort, the anonymity of the occupancy data meant that the identities of these students could only be inferred; ethnographic observations clarified the apparent identities and activities of users, while field interviews enabled us to confirm these demographic details for key instances. Ethnographic observation and interviews in the adjacent breakout space confirmed that, in general, most students were waiting and preparing for the timetabled lecture during the on-ramp transition period and reflecting and planning together during the off-ramp transition period. This qualitative data collection hence supported our characterisation of the breakout space as an area of pedagogical potential proximal to the lecture theatre. Being equipped with comfortable furniture and amenities better-supported students in their engagement with this potential during on-ramp and off-ramp transitions and associated learning activities.
The automated recording of occupancy data enabled the analysis of occupancy patterns across the expected, timetabled working week for 6 weeks with 14 days (120 h) data studied in more detail to confirm and further investigate the type 1 and type 2 timetable configurations. Subtracting lecture theatre and breakout space occupancy during timetable crossover periods provided data from which averages could be calculated for the percentage of year 1 or year 2 cohorts remaining and engaging with transitions in the breakout space. The average percentages provide insight into the potential influence of the timetable configuration on whether students were more or less likely to use the adjacent breakout space during transition periods. Based on the arithmetic mean, more (43%) of the students meaningfully engaged in the off-ramp transition during the ‘bridge’ space following the 1st year lecture in the type 2 timetable, whereas less (19%) of the students meaningfully engaged in the off-ramp transition in the type 1 timetable (see Fig. 1 ). The type 1 configuration seemed to provide less incentive for 1st year students to remain in the breakout space beyond their 9:00–11:00 lectures given that their timetabled expected interactions were not in that space. This was confirmed in field interviews, for example, a 1st year chemical engineering student was observed leaving her 10:00–11:00 chemistry lecture and explained how she typically used the breakout space:
“I usually tend to just quickly go over stuff, often with friends…purely because of its location and proximity to the lecture theatre”.
This behaviour of quickly reviewing material was mirrored during observation of other students leaving the same lecture 10 min prior, two of whom were overheard sharing “I kind of lost it after…” and “Did you understand…” as they stood to leave the lecture theatre just after the session had finished. While two students were observed asking the lecturer questions at the front of the lecture theatre, all but three immediately exited the lecture theatre and breakout space, likely heading to the campus library, to buy lunch or return to their hall accommodation.
In the type 1 timetable, 20% of the students meaningfully engaged in the on-ramp transition in the breakout space prior to their 2nd year lecture, whereas more (52%) of the 1st year students engaged in the on-ramp transition in the type 2 timetable (see Fig. 1 ). These statistics further support the potential of the ‘bridge’ period which might be understood as the interaction between the 1st year off-ramp and on-ramp transition periods in the type 2 timetable configuration. Ethnographic observation of student engagement within this bridge period revealed tensions between the expected timetabled teaching activity and more self-directed non-timetabled space and/or time. While occupancy analysis provided cohort-level insight into the spatial transitions within this bridge space and/or time (see Fig. 2 for example), observations aided in the discovery of less obvious complexities, such as tensions and potential within and between spaces and/or time. Field interviews with students deepened this understanding by confirming differences in student intent during this bridge time to either remain in the lecture theatre or breakout space ahead of the second lecture or migrate elsewhere to buy coffee or lunch, for example. Two 1st year chemical engineering students who departed their first lecture just before 11:00 (at the start of a bridge period) were observed moving together to a sofa in the breakout space. Overhearing their conversation, one admitted “The one thing I didn’t get…” to the other, who responded by confessing “How did he go from these three to these…” whilst pointing at paper notes. This observation strongly suggested that the two students were discussing difficult content from their fluid mechanics lecture, which was confirmed in a field interview in which they explained “Yes we were discussing concepts from the lecture” and that they “use this space between lectures”, stating their reason for using it as:
“It is purely because of the convenient location being by our lectures and that it is quite quiet. It is also quite spacious”.
The bridge period on this same day was dissimilarly used by a group of five 1st year students from the same cohort who chose to remain in the lecture theatre until around 11:26. Whilst they opted to use the lecture theatre instead of the breakout space, they seemed to engage in similar behaviour by clarifying misunderstandings with each other, before leaving the space ahead of their next lecture at 12:00. These findings suggest that the type 2 configuration may have the potential for more meaningful transition engagement than the type 1 configuration by providing 1st year students (who planned to attend both lectures) with a ‘bridge’ 11:00–12:00 containing 1 h of self-directed pedagogic potential sandwiched between 2 h of formal teaching contact.
Further understanding how these timetable configurations create the potential for connection and transition between timetabled activities might lead to alternative ways of informing timetable and instructional design and supporting active learning. While the mixed methods approach assisted in understanding transitions and tensions, a deeper understanding of this dynamic system eventually became limited by the language and concepts adopted. Taking an ecological approach helped us to overcome this limitation by enabling us to broaden the ethnographic focus on spatial transitions and tensions to also include those concerning pedagogy and agency. Furthermore, this approach crucially led to thinking about spaces not only as separate ecosystems but also as interconnected and integrated ecological zones within which there is different potential.
Pedagogic transitions and tensions
Conceptualising the lecture theatre and breakout space as part of an interconnected ecological zone with on-ramp and off-ramp transitions provided an alternative way of thinking about the flows of people and information between the timetabled and non-timetabled periods. Ethnographically observing these transitions provided more nuanced insight into the tensions and student behaviours typical of these periods. The physical configuration, timetabled intention and historic usage of the lecture theatre created fixed patterns of expected behaviour, which centred around transmission-based approaches to teaching and learning where the teacher typically stands at the front and lectures to students who absorb information in the row-by-row seating area. The fixed power dynamic of the lecture theatre can often suppress student interaction and result in ‘failed’, hidden, and postponed pedagogic interactions 8 . ‘Failed’ pedagogic interactions are characterised by periods in which students with internal confusion feel disempowered and disinclined to raise their hand and ask questions, even when invited to do so by the lecturer. In instances where students ask a question, the lecturer may often simply provide an answer and, in so doing, maintain the expected flow of transmitted information and reinforce the power dynamic. While some lecturers occasionally deviate from this pedagogic style by, for example, encouraging class discussion using real-time surveys, they often feel limited by the inherent expectation of the physical space.
By choosing not to volunteer misunderstandings in front of the class during such ‘failed’ pedagogic interactions, some students can instead undergo hidden pedagogic interactions. These can be observed as brief periods of whispering and question exchange between neighbouring students or as students consulting personal technology during the lecture. They are termed ‘hidden’ because they do not explicitly conform to transmission-based pedagogic expectations and are often actively discouraged by the teacher who commands students’ attention. One lecturer was observed responding to this type of student interaction in a 1st year lecture by telling the whole class to “please listen carefully, this is extremely important” and further reinforcing the expected dynamic by responding to perceived distraction with “no talking please”.
Other students may respond differently to their internal confusion by ‘postponing’ their questions to the end of the lecture. Some students undergoing these postponed pedagogic interactions during an off-ramp transition were observed gathering at the front of the lecture theatre to question the lecturer individually and more privately. Furthermore, the sociogram in Fig. 3 typifies group learning behaviour observed during an off-ramp transition period in the breakout space. In this case, the sociogram was recorded as soon as students left a 2nd year lecture timetabled until 16:00 to observe how members of the cohort engaged with the transition between the lecture theatre and adjacent space. The sociogram was recorded on a day configured with a type 2 timetable during an off-ramp transition much like the final one labelled on the occupancy plot in Fig. 2 (for a different day). As seen in the sociogram, a large group of eight students was observed engaging in a postponed pedagogic interaction in which they left the lecture theatre and immediately organised themselves around a table in the breakout space. The size of the group meant it was inappropriate to approach them for a field interview, yet their conversational tone and behaviour suggested they were discussing something work-related and possibly in relation to a “project”. The sociogram helped us to identify and approach a different chemical engineering student for a field interview in the corner of the breakout space who had also left the 2nd year lecture. She shared that she used the breakout space “because of its location” and that it is “quite comfortable because of the furniture”. This supports the view that the presence of such amenities and affordances outside of the lecture theatre can support students’ postponed pedagogic interactions.
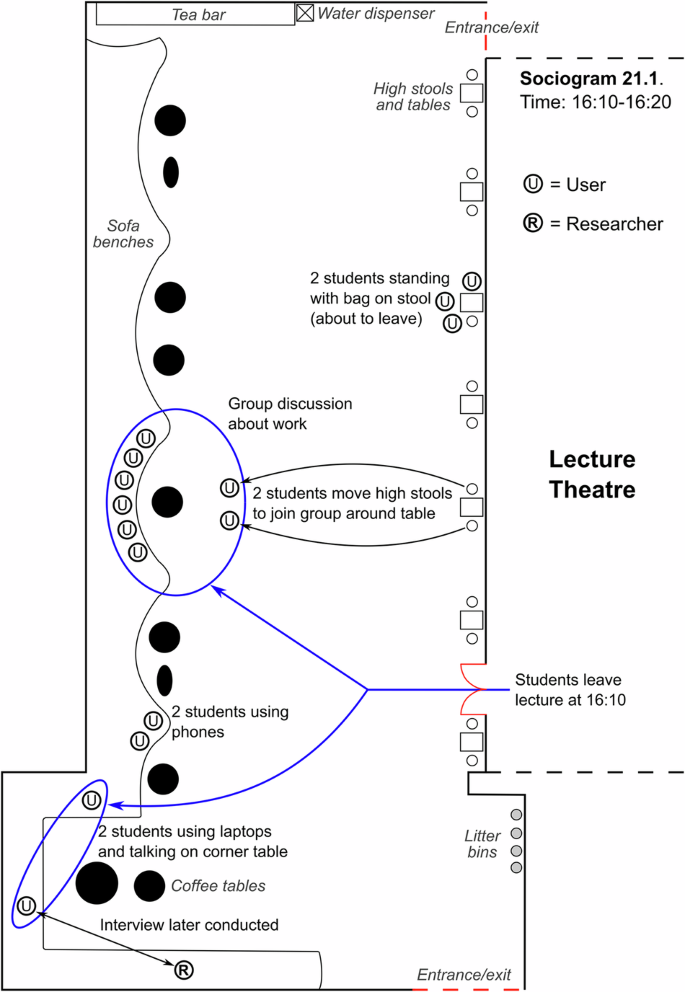
Digitised sociogram of the breakout space showing user behaviour and interaction during an off-ramp transition immediately after a 2nd year lecture in the adjacent lecture theatre.
As both teachers and students are subject to power dynamics and associated expectations that promote the transmission/absorption of information during timetabled learning, passive transmission-based learning is often perceived as a lower-risk activity than more student-centred active learning. In suppressing certain pedagogic potentials, a ‘pedagogic tension’ exists, within which students can sometimes manage the tension and more freely exercise their pedagogic intent. These pedagogic potentials were observed as ‘failed’, hidden and postponed pedagogic interactions, the behavioural signatures of which were visualised as sociograms (such as in Fig. 3 ) and confirmed using field interviews. Conceptualising this pedagogic tension between traditional passive learning and active learning as an ecological phenomenon changed how we thought about the space between the lecture theatre and the breakout space, while also recognising more broadly the opportunities for strategic changes in pedagogic mindset and behaviour.
Agentic transitions and tensions
Investigating how agency and ‘ownership’ transitioned between the timetabled lecture theatre and non-timetabled breakout space and/or time periods aided in obtaining a better understanding of the conditions needed for inclusive active learning. Transmission-based teaching created pressure for students to experience ‘failed’, hidden and postponed pedagogic intent. On-ramp and off-ramp periods were ethnographically determined to be important spaces for supporting these transitions and managing tensions, as well as key sites of strategic change. Field interviews confirmed that students perceived less ownership of the lecture theatre than the teacher, who predominantly controlled the lecture interaction. Students also confirmed that they perceived the adjacent breakout area as a more democratic space, including one student who explained how they “can talk here and make some noise” whilst feeling “free to do anything”. In informal conversations, teachers concurred that they found it helpful to answer one-on-one student questions in a more informal setting like the breakout space.
The fixed power dynamic and potential fear of judgement often implicit in ‘typical’ lecture theatre interactions seemed to emphasise a more democratic power dynamic in the breakout space, such that students felt emancipated to direct questions and take more risks in their learning in this adjacent space. Student-directed questioning of peers and the teacher, as they physically moved from the lecture theatre into the breakout space during off-ramp transitions, suggested a renewed sense of agentic ownership in their learning. The affordances and flexibility of the breakout space meant that students under their own control could form pairs and small learning groups more easily than in the row-by-row lecture theatre. Ethnographic observations confirmed that this apparently incidental and procedural activity resulted in important dialogue and sharing between students, their peers and the teacher. The ‘agentic tension’ between the teacher-owned lecture theatre and the student-owned breakout space might be understood more broadly as an important tension between the historic way of learning traditionally in a lecture theatre and the pedagogic intent for learners to discover and explore via more active learning. Understanding this agentic tension also led to new ways of considering/managing the pedagogic balance between teacher-centred transmission and student-centred independent learning; this potential balance is dynamic and different for every student, cohort and teacher.
The public nature of the breakout space also led to the possibility of tensions between different user groups who perceived differing ownership of the space. These tensions changed throughout the academic year—based on who frequented the adjacent lecture theatre and surrounding spaces—and influenced the perceived agency of different users. The lecture theatre predominantly served early-year undergraduate students, who, as a function of their habitual ingress and egress via the breakout space, somewhat resulted in cohort familiarity and feelings of ownership; other research has shown that the intensity of these feelings can increase with the time spent there 26 . Studying on-ramp and off-ramp transitions and tensions between the two spaces provided insight into how different user groups interacted and vied for space ownership depending on their planned or unplanned activity. Later-year undergraduate students, for example, reported a change in their use of the breakout space (since their time as early-year undergraduates) based on their changed location of timetabled activities. This can be demonstrated by the field interview response of a 3rd year chemical engineering student who was interviewed with her friend in the breakout space:
“I like departmental spaces because of convenience and because of the micro community from being around other departmental members and friends. However, I would also say the way my friends and I use departmental spaces has changed over the course of our degree, as we now use different spaces to 1st year students, for example”.
Similarly, postgraduate students confessed to a greater sense of ownership during the summer months when undergraduate students no longer had timetabled teaching. This is exemplified by the interview response of a PhD student in chemical engineering who described the breakout space as:
“Not a place for work, because the undergraduates are here—sometimes I can’t even come here to eat because it is so full. Undergraduates tend to do group work here a lot as they have nowhere else.”
These agentic tensions therefore governed the sense of ownership different user groups felt in the space throughout the academic year and influenced how these users could enact certain activities. Framing these dynamic and temporally changing agentic tensions as ecological phenomena supported a deeper understanding of their nature and impact on student pedagogic interactions.
This paper has used data from a mixed methods study of student pedagogic interactions in a traditional lecture theatre and adjacent transitional space to illustrate the potential of an ecological approach, when investigating complex transitions and tensions between timetabled and non-timetabled learning spaces in a STEM university context. Three different categories of transitions and tensions between these spaces have been defined: ‘spatial transitions and tensions’, which exist as a function of timetables and architectural divides between formal and informal space and the associated expectation to learn in the formal timetabled space and/or time; ‘pedagogic transitions and tensions’, which exist between teacher-centred transmission intent within a space and student-centred intent, which can result in ‘failed’, hidden and postponed pedagogic interactions where students negotiate their own space for tackling misunderstanding; and ‘agentic transitions and tensions’, which occur between the fixed power dynamic of the formal lecture theatre and the more democratic nature of the adjacent informal space, resulting in differing agentic ownership between students and teachers in the different learning spaces.
Initially thinking about the lecture theatre and breakout space as fixed, isolated entities presented a barrier to understanding these complex interactions and tensions. We postulate that the ecological concept of the ‘ecotone’ may significantly advance our understanding of complex interactions between space and pedagogy in transition and tension. From an ecological perspective, ecotones not only represent zones of overlap and transition—for example, in estuarine intertidal zones between ocean and river ecosystems—but also exist as a distinct third ecosystem subject to conflicting tidal and river forces which create tensions and alter its position and makeup 27 . The increased biodiversity of edge species within an ecotone, which translates etymologically to mean ‘ecologies in tension’, emerges from the opportunities afforded by two ecosystems coexisting in an interconnected and dynamic way. By being less encumbered by the inertia and rules of the adjoining spaces, ecotones present opportunities for transition and potential evolutionary innovations that feedback into the core 28 . Although the use of the ecotone concept in higher education is limited 27 , 29 and presents challenges by applying a metaphor from nature to model social systems 30 , its application in our research suggests its potential to transform our understanding of complex phenomena within evolving university systems.
Adopting an interrelated, dynamic view of the complex context of learning in a changing situation is necessary, given a rapidly changing context within which increasingly diverse models of learning can have a specific educational meaning. Although this paper has introduced the ecotone concept as a way of thinking about how space, pedagogy and agency interact and how tensions between them inhibit or enable learning, there may be other potential applications of the ecotone. For example, the concept could help us to think about a diverse group of students, each with individual cultural and learning expectations; different initial knowledge and skills; and different strategies, approaches and goals working together. Managing possible tensions within these groups while embracing a ‘biodiversity’ of people and ideas can become an important source of system resilience 31 . Perceiving this diversity as a source of positive pedagogic potential can inform the design of timetables and spaces for more productive active learning.
With the World Economic Forum anticipating the ‘metaverse’ as one of the top ten emerging technologies 32 , it is arguably important to think about how this new ‘in-between’ reality will enhance connectivity between people. For example, there has been a recent move away from dualistic definitions of online and physical space to ‘onlife space’ 33 , which encapsulates both the physical and virtual realms and acknowledges the fundamental role of information technology in changing and activating physical spaces. The ecotone might be a credible metaphor for conceptually framing these onlife spaces, helping to acknowledge transitions and tensions that exist between reality and virtuality; the concept has separately been positioned as a useful metaphor for conceptualising the ‘…third space at the intersection of analogue, digital, and postdigital learning spaces’ 29 .
With the institution’s desire to increase active learning pedagogy, our findings encouraged a reflection on the extent to which existing learning spaces and timetabled pedagogic interactions were always appropriate for students to navigate transitions between traditional transmission-based learning and more active learning. Applying the ecotone metaphor first helped with recognising the formal and informal spaces as greater than the sum of their parts, which facilitated a more nuanced data analysis and wider institutional discussion that raised awareness of tensions. It also subsequently evidenced the redesign of several lecture theatres, with the intent of providing spaces better designed to facilitate more flexible pedagogic and agentic transitions within both timetabled and non-timetabled periods. However, redesigning traditional lecture theatres to be more architecturally aligned with active learning classrooms 7 is time- and cost-intensive and will mostly empower local changes to teacher and student agency. The already disproportionate capital investment into UK university education space, exceeded only by staff budgets, renders a broader redesign of all campus lecture theatres difficult 22 .
By instead using a mixed-method ecological approach to highlight the pedagogic potential of informal transitional spaces that exist between these more formal spaces and interactions, ecotone thinking can empower teachers to reconsider their use of existing spaces such as the lecture theatre and breakout space introduced in this paper. For instance, inviting students to move between such spaces can aid group formation and/or transitions between transmission-based lecture segments and more active group-based interactions. The understanding gained from these incidental and purposeful transitions between formal and informal spaces informed a series of student-staff partnership projects that redesigned ‘transitional spaces’ just outside of lecture theatres 10 . The ecological thinking therefore resulted in a ‘regenerative design’ approach 34 in which spaces were redesigned more cost-effectively, teachers could evolve their perceived and actual use of space and students could better connect their timetabled learning to various contexts.
The sector-wide shift from an industrial model of universities towards an ecological model 14 could itself be thought of as an ecotone within which students, teachers, policymakers, administrators and leaders operate and negotiate change. The ecotone concept may be valuable as a framework for considering complex, dynamic situations in which two or more things interact with the possibility of tensions existing between them. Higher education institutions that embrace an ecological mindset might be better prepared to navigate disruptions, whether they stem from technological advancements, shifts in student demographics, or global events such as the COVID-19 pandemic. The goal might be to move from a transactional to a transformative view of higher education 29 , which better supports the development of an ‘autonomous lifelong, life-wide learner, a capable knowledge worker, and a critical citizen’ 24 who has the capacity to change, learn and tackle the wicked challenges that the world faces.
Settings and participants
This research aimed to explore the nature of transitions within and between a ‘traditional’ lecture theatre used for timetabled activity and an adjacent informal breakout space (see Fig. 4 floorplan) in the institution’s chemical engineering department. This research setting was selected because of the interesting tension in activity between the lecture theatre—a row-by-row space with a 160-seat capacity serving 1st and 2nd year undergraduate classes of 30–150 students—and the public nature of the breakout space, used freely by a diverse group of users from inside and outside the department. This tension made the setting ideal for exploring transitions and potentials between different types of learning activities. The configuration and pedagogical use of the lecture theatre exemplified the ‘status quo’ across the STEM institution at the time of data collection. This provided a useful starting point for understanding existing learning behaviours and transitions amid the institution’s strategic transformation of learning space, curriculum and pedagogy. Furthermore, studying this setting was opportune following the institution’s investment in occupancy monitoring technology, which generated anonymous occupancy data for both spaces. The occupancy technology automatically collected data 24 h per day, 7 days per week, all year round allowing us to identify occupancy patterns and transitions and target specific days and times of interest. The wider, longer-term occupancy dataset was initially analysed at a higher, less granular level to select an appropriate setting for the research. Once the lecture theatre and breakout space had been selected, around 120 h of occupancy data spanning two academic years were analysed in more granular detail to investigate cohort transitions between the learning spaces, including for different timetable configurations.
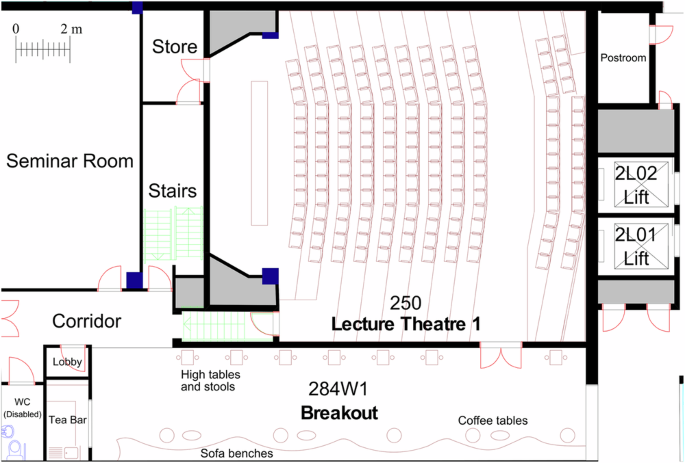
Breakout space at the base shows a variety of furniture types and entrances to other spaces, including the traditional lecture theatre above with raked row-by-row seating for transmission-based teaching.
This occupancy data informed our subsequent targeting of data collection resources when employing the qualitative methods 25 , enabling a more efficient and purposeful targeting of observations. We conducted 30 observations on 29 separate weekdays within 22 weeks across a period of 11 months. Given observations lasted between 30 min and 90 min, depending on if timetabled lecture sessions had also been observed, observations totalled around 24 h and were always conducted within the confines of the academic day (09:00–17:00) and term time. Straight after some observations, we also conducted a total of 21 field interviews with 25 student participants (as some interviews involved more than one participant).
Participants were purposefully sampled for field interviews based on their observed learning behaviours during transitions between timetabled and non-timetabled spaces and periods. As these field interviews were designed to be brief (5–10 min) and non-disruptive, we retrieved the degree type and year of study from participants, opting to note other demographic details based on our observation to avoid taking too much time. Of the 25 field interview participants, close to 50% were from the 1st and 2nd year undergraduate chemical engineering cohorts observed in lecture sessions; 4 interview participants were students from other departments and 4 participants were studying postgraduate degrees, demonstrating the user diversity of the breakout space. For a notional 40-h study week, the early-year undergraduate cohorts were expected to attend teaching contact for 25% of their time, with the remaining 75% of the time being spent independently studying. This emphasis on informal work is dictated by the degree assessment, which in the early years consisted of 10% practical, 20% coursework and 70% examination. The curricular requirement for formal and informal learning in this degree context made the transitions between timetabled and non-timetabled learning particularly interesting, and typical of many other STEM subjects taught at this institution.
A mixed method for understanding transitions and tensions
The combination of quantitative space occupancy data with qualitative insights from ethnographic observations and field interviews led to a deeper understanding of how students perceive and engage with the learning spaces and the transitions between them 25 . The wider, longer-term occupancy dataset was initially analysed at a higher, less granular level for various spaces across the campus to identify potentially interesting settings in which qualitative data collection might be targeted; our secondary use of this occupancy data deviated from its intended institutional purpose of optimising room bookings and space efficiency. Occupancy insights for the lecture theatre and breakout space helped to reveal the ecological nature of the learning environment with inflow, outflow, transition and dwelling of people within and between the spaces. The occupancy data also guided the more resource-intensive targeting of ethnographic observations and field interviews which allowed us to interpret the meaning and nuance of those transitions and possible associated tensions.
‘Naturalistic’ ethnographic observation protocols 35 were chosen because they are non-participant and minimise the chances of participants altering their behaviour; as a younger researcher, I was able to remain inconspicuous as an observer. Observations were recorded as field notes on a laptop and were sometimes supplemented with floorplan-based sociograms which captured person-person and person-space interactions within 10-min snapshots of breakout space activity (see Fig. 3 for example). Sociograms were mostly recorded during timetable transition periods (such as immediately after a lecture when students would flow into the breakout space) and helped us to target field interviews by visualising the broader context, identifying specific individuals or small groups who exhibited interesting learning behaviour whilst being sensitive to who we approached. Field interviews were initiated with verbal consent due to the informal low-risk nature of interviews, which posed structured questions pertaining to how exactly participants were using the space (as compared to the observation), how often they typically used the space, why they chose to use this specific space and where else they would typically carry out the same activity on campus. Informed consent was not used for these brief in situ field interviews, a decision approved as part of two detailed applications (reference numbers EERP1718-021 and EERP1819-012) made to Imperial College’s Education Ethics Review Process. The ethics committee agreed that using informed consent protocols for 5–10-min field interviews posed an inappropriate time cost for participants, whilst potentially affecting the quality of collected data by distancing it from the observed behaviour. Retrieving brief verbal consent posed less risk and was arguably more commensurate with the uncontentious informal nature of questioning.
Thematic analysis 36 was used to analyse the ethnographic observation and field interview data by developing ‘themes’ in the qualitative data. These themes are patterns of shared meaning unified by a central organising concept, which emerge inductively from drawing relationships between different segments of the data that are assigned smaller units of meaning called codes. Analysis began during the collection of ethnographic field notes given these inscriptions were shaped by what I ‘saw’ and the choices I made as an ethnographer. To minimise bias, we remained reflexive and used our positioning and contextual knowledge, including when looking at patterns in the objective occupancy data or when using those results to target subsequent observations, to repeatedly make meaning of the data and develop a critical perspective 20 . Observation and field interview data were separately analysed in NVivo software to develop codes and themes which could then be understood holistically.
The mixed method approach and analysis of that data embodied ecological principles by dynamically and reflexively moving between remote analysis of cohort-level occupancy patterns and in situ observations and field interviews. This triangulation supported a more complete understanding of phenomena and a more resourceful collection of authentic data. The use of this approach in other settings in the institution has informed the development of infrastructure, spaces and places in which transitions and tensions are better managed and learning ecologies are able to organically develop.
Reporting summary
Further information on research design is available in the Nature Research Reporting Summary linked to this article.
Data availability
Some of the data collected in the research study is available, as limited by ethical approval, and can be requested by contacting the corresponding author (L.M.).
Ballen, C. J., Wieman, C., Salehi, S., Searle, J. B. & Zamudio, K. R. Enhancing diversity in undergraduate science: self-efficacy drives performance gains with active learning. CBE Life Sci. Educ. 16 , ar56 (2017).
Article PubMed PubMed Central Google Scholar
Freeman, S. et al. Active learning increases student performance in science, engineering, and mathematics. Proc. Natl. Acad. Sci. USA 111 , 8410–8415 (2014).
Article CAS PubMed PubMed Central Google Scholar
Vygotsky, L. (ed) Mind in Society: The Development of Higher Psychological Processes (Harvard University Press, 1980).
Eison, J. & Bonwell, C. (eds) Active Learning: Creating Excitement in The Classroom (George Washington University, 1991).
Imms, W. & Kvan, T. (eds) Teacher Transition into Innovative Learning Environments: A Global Perspective . (Springer Nature, 2021).
Beichner, R. J. et al. The student-centered activities for large enrollment undergraduate programs (SCALE-UP) project. In: Research-Based Reform University Physics (American Association of Physics Teachers, 2007).
Beichner, R. J. History and evolution of active learning spaces. N. Dir. Teach. Learn. 2014 , 9–16 (2014).
Article Google Scholar
McCrone, L. (ed) Transitional Space in Active Learning: Perspectives From an Undergraduate Stem Education Context (Imperial College London, 2021).
Harrop, D. & Turpin, B. A study exploring learners’ informal learning space behaviors, attitudes, and preferences. N. Rev. Acad. Librariansh. 19 , 58–77 (2013).
Streule, M., McCrone, L., Andrew, Y. & Walker, C. Engaging with students as partners in education-space design. Int. J. Stud. Partn. 6 , 79–90 (2022).
Google Scholar
Howell, L. L. & Bateman, T. Extending research impact by sharing maker information. Nat. Commun. 14 , 6170 (2023).
Walker, B. & Salt, D. (ed) Resilience Thinking: Sustaining Ecosystems and People in a Changing World (Island press, 2012).
Barnett, R. (ed) The Ecological University: A Feasible Utopia (Routledge, 2017).
Kinchin, I. M. Five moves towards an ecological university. Teach. High. Educ. 28 , 918–932 (2023).
Sobel, D. Place-based education: connecting classrooms and communities. Educ. Mean. Soc. Justice 17 , 63–64 (2004).
Stauffer, R. C. Haeckel, Darwin and Ecology/RC Stauffer. Q Rev. Biol. https://doi.org/10.1086/401754 (1957).
Bateson, G. (ed) Steps to an ecology of mind: collected essays in anthropology, psychiatry, evolution, and epistemology (University of Chicago Press, 1972).
Bronfenbrenner, U. (ed) The Ecology of Human Development . (Harvard University Press, 1979).
Bronfenbrenner, U. & Morris, P. A. The bioecological model of human development. in Handbook of Child Psychology (John Wiley & Sons, Inc., 2007). https://doi.org/10.1002/9780470147658.chpsy0114 .
Madden, R. (ed) Being Ethnographic: A Guide to the Theory and Practice of Ethnography (Sage, 2017).
Barnett, R. & Jackson, N. Ecologies for Learning and Practice: Emerging Ideas, Sightings, and Possibilities (Routledge, 2019).
Temple, P. University spaces: creating cité and place. Lond. Rev. Educ. 17 , 223–235 (2019).
Browndorf, M. Student library ownership and building the communicative commons. J. Libr Adm. 54 , 77–93 (2014).
Goodyear, P. & Ellis, R. A. Ecological thinking about education strategy in universities. In Ecologies for Learning and Practice: Emerging Ideas, Sightings, and Possibilities (eds. Barnett, R. & Jackson, N.) 97–111 (Routledge, 2019).
McCrone, L. & Kingsbury, M. Combining worlds: a mixed method for understanding learning spaces. Int. J. Qual. Methods 22 , 16094069231173780 (2023).
Sommer, R. (ed) Personal Space: The Behavioral Basis of Design (Prentice-Hall, 1969).
Pendleton-Jullian, A. Education and Innovation Ecotones. in Ecologies for Learning and Practice: Emerging Ideas, Sightings, and Possibilities (eds. Barnett, R. & Jackson, N.) 112–128 (Routledge, 2019).
Hagel, J., Hagel III III, J. & Brown, J. S. The Only Sustainable Edge: Why Business Strategy Depends on Productive Friction and Dynamic Specialization (Harvard Business Press, 2005).
Ball, J. & Savin-Baden, M. Postdigital learning for a changing higher education. Postdigital Sci. Educ. 4 , 753–771 (2022).
Ghazinoory, S., Phillips, F., Afshari-Mofrad, M. & Bigdelou, N. Innovation lives in ecotones, not ecosystems. J. Bus. Res. 135 , 572–580 (2021).
Buschke, F. & Brownlie, S. Reduced ecological resilience jeopardizes zero loss of biodiversity using the mitigation hierarchy. Nat. Ecol. Evol. 4 , 815–819 (2020).
Article PubMed Google Scholar
World Economic Forum. Top 10 Emerging Technologies of 2023 (World Economic Forum, 2023).
Floridi, L. (ed) The Onlife Manifesto: Being Human in a Hyperconnected Era (Springer Nature, 2015).
Cole, R. J., Oliver, A. & Robinson, J. Regenerative design, socio-ecological systems and co-evolution. Build. Res. Inf. 41 , 237–247 (2013).
Somekh, B. & Lewin, C. (eds) Research Methods in the Social Sciences (Sage, 2005).
Braun, V. & Clarke, V. Using thematic analysis in psychology. Qual. Res. Psychol. 3 , 77–101 (2006).
Download references
Acknowledgements
We thank those involved in the development and execution of the institutional strategy that funded and motivated this research. We also thank fellow members of the Centre for Higher Education Research and Scholarship for their input into the thinking behind the research. The open access fee was paid from the Imperial College London Open Access Fund. Finally, we thank all the participants who contributed valuable insights and experience during the data collection.
Author information
Authors and affiliations.
Centre for Higher Education Research and Scholarship, Imperial College London, London, UK
Luke McCrone & Martyn Kingsbury
You can also search for this author in PubMed Google Scholar
Contributions
L.M. collected and analysed the data and wrote the first draft of the paper. M.K. secured the research funding, helped to analyse the data, and coauthored the paper.
Corresponding author
Correspondence to Luke McCrone .
Ethics declarations
Competing interests.
The authors declare no competing interests.
Additional information
Publisher’s note Springer Nature remains neutral with regard to jurisdictional claims in published maps and institutional affiliations.
Supplementary information
Editorial policy checklist, rights and permissions.
Open Access This article is licensed under a Creative Commons Attribution 4.0 International License, which permits use, sharing, adaptation, distribution and reproduction in any medium or format, as long as you give appropriate credit to the original author(s) and the source, provide a link to the Creative Commons licence, and indicate if changes were made. The images or other third party material in this article are included in the article’s Creative Commons licence, unless indicated otherwise in a credit line to the material. If material is not included in the article’s Creative Commons licence and your intended use is not permitted by statutory regulation or exceeds the permitted use, you will need to obtain permission directly from the copyright holder. To view a copy of this licence, visit http://creativecommons.org/licenses/by/4.0/ .
Reprints and permissions
About this article
Cite this article.
McCrone, L., Kingsbury, M. An ecological approach to understanding transitions and tensions in complex learning contexts. npj Sci. Learn. 9 , 54 (2024). https://doi.org/10.1038/s41539-024-00267-1
Download citation
Received : 16 February 2024
Accepted : 15 August 2024
Published : 06 September 2024
DOI : https://doi.org/10.1038/s41539-024-00267-1
Share this article
Anyone you share the following link with will be able to read this content:
Sorry, a shareable link is not currently available for this article.
Provided by the Springer Nature SharedIt content-sharing initiative
Quick links
- Explore articles by subject
- Guide to authors
- Editorial policies
Sign up for the Nature Briefing newsletter — what matters in science, free to your inbox daily.

- Open access
- Published: 09 September 2022
A review of landscape ecology experiments to understand ecological processes
- Yolanda F. Wiersma ORCID: orcid.org/0000-0003-4604-9240 1
Ecological Processes volume 11 , Article number: 57 ( 2022 ) Cite this article
5492 Accesses
4 Citations
1 Altmetric
Metrics details
One way in which we make inferences about ecological processes is via experimentation. Many ecological processes happen at landscape extents and it is at this extent that experimentation is more challenging. This review explores the intersection between experimentation, ecological processes and landscape ecology. Specifically, this review seeks to discover how scientists design experiments to understand ecological processes at landscape scales.
I found 87 papers where these three concepts intersected, and reviewed them in more depth to assess characteristics of scale (treatment and study area extent), replication, research question and experiment type.
Conclusions
The findings suggest that experimental approaches for understanding ecological processes are well established, and beginning to more readily accommodate spatial dimensions. However, there is room to integrate more spatially explicit, landscape-scale experiments into studies of ecological processes.
Introduction
A key tool for understanding mechanisms that shape patterns is via experimentation. This is true across scientific disciplines. Ecological processes, the focus of this journal, shape and influence ecological systems at all scales. Although research in this journal has traditionally examined ecological process at many different extents, ecological processes at large extents merit special consideration since these shape the systems that humans directly interact with, actively manage, and critically depend on. These include the agricultural and marine ecosystems that feed us, the forest ecosystems that provide timber and non-timber resources, and the myriad of ecosystems that provide carbon sequestration. These large extent systems of fields, forests and oceans are also the purview of the discipline of landscape ecology (Turner 2005 ; Turner and Gardner 2015 ). How to carry out experiments in landscapes to realize reliable inferences about the links between ecological patterns and ecological processes, and vice versa, is a key challenge for researchers, and is the focus of this review.
In a perspectives essay on experimental landscape ecology, Jenerette and Shen ( 2012 ) discussed different experimental approaches to identify how landscapes affect variation in ecological processes, and how landscape structure influences these processes. They highlighted the challenge of carrying out experiments at landscape extents (generally 10–100 s of kilometres), citing difficulty with replication, and the complexity of setting up experiments in spatially heterogeneous systems (Jenerette and Shen 2012 ). Many landscape-scale studies rely on observational data, and rely on correlations to infer processes, which may not capture the actually mechanisms at play.
Jenerette and Shen ( 2012 ) suggested four types of experiments that landscape ecologists could apply to help identify process variation within a landscape. These include distributed in situ experiments; ex situ experiments using samples collected throughout a landscape and brought back to the lab for analysis, translocation experiments and transport manipulations. Their group of experiment types to identify how processes responded to landscape structure echo many of the “classic” large-scale experiments, such as the experimental patches at the Savannah River Ecosystem, the Bowling Green fragmentation experiment, or systems such as Ecotrons (see an excellent summary of these types of experiments in Haddad 2012 ). Such experiments manipulate patch shape, connectivity, and fragmentation. Other experiments that can be used to infer how landscape structure affects process include manipulation of internal patch characteristics (e.g., via adding artificial structures, or adding nutrients), manipulation of landscape scale (e.g., mesocosms, microcosms, microlandscapes) or the construction of entire landscapes (Jenerette and Shen 2012 ). Wiersma ( 2022a , b ) summarized these approaches (large-scale manipulations, mesocosms, microcosms) along with in silico experiments (i.e., computer models) in more detail to show how researchers could harness these experimental tools to do spatially explicit experimentation (See Box 1 ). In this review, I pay particular attention to the experimental types (according to the taxonomy in Jenerette and Shen 2012 ) and tools used to study ecological processes at landscape extents.
Before discussing the literature review in more detail, a review of key terms is necessary. There has been much debate about whether we should consider observational studies to be “proper experiments” or not (Diamond 1983 ). In the mid-twentieth century, the increased reliance on sophisticated technology in the bench sciences, particularly molecular genetics, suggested to some ecologists that their field observational studies were too close to amateur natural history studies to be considered experimental and that manipulative experiments were the more reliable means for testing hypotheses (Kohler 2002 ). To clarify, by a manipulative experiment here, I mean an experiment whereby the researcher actively manipulates a factor of interest. This could be at a large extent in the field (e.g., via a controlled burn), in the field at smaller extent (e.g., via exclosures) or in a laboratory setting (e.g., experimental tanks or aquaria under controlled environments). Observational experiments (also called “natural experiments”) are those where natural processes have created the experimental treatment conditions. This treatment could be in the form of a disturbance, such as a flood or forest fire, or could be due to an underlying natural gradient (e.g., topography, soil moisture, light levels). If sampling is carried out as carefully as possible, so that experimental standards of control, replication, and randomization are applied, many suggest that observational experiments should be considered an equally credible approach as a manipulative one (Diamond 1983 ). Indeed, Diamond ( 2001 ) points out that heading to the field with a too narrowly focused experiment in mind can risk missing the chance to carry out an unplanned natural experiment. Laboratory/manipulative experiments have advantages of being easier to control for confounding effects, but being less realistic. Field manipulative experiments are more realistic, but can be logistically challenging to implement and have limited replication, and be influenced by stochastic events at the particular point in space and time they are implemented. Thus, it can be more challenging to meet the standards of experimental design in a manipulative field experiment (Diamond 1983 ; Wiersma 2022a ). Observational experiments are the most realistic, but the experimenter loses control over every aspect of the study except where and when they sample. For the purposes of this review, I am considering both observational (“natural”) and manipulative approaches in my consideration of what is an experiment. Moreover, this review is limited to experiments in ecological science. While studies of ecological processes and research in landscape ecology can certainly benefit from integration of methodology from the social sciences, an assessment of methodological approaches in social science is outside the scope of this review.
The experimental aspects of a control (a set of observations identical to the experiment minus the treatment factor), and randomization (ensuring experimental treatments and/or sampling are carried out without bias to underlying conditions) should be familiar to scientists. Conceptually, they are straightforward, but when working at large landscape extents, it can be difficult to implement these (Jenerette and Shen 2012 ; Wiersma 2022a , b ). The issue of replication can cause more confusion. Replication can happen at the experimental unit and at the sampling unit, and sometimes researcher can be confused as to what their sample size actually is. An experimental unit is defined by Krebs ( 1989 : 269) as “the smallest division of the experimental material such that any two units may receive different treatments”. A sampling unit, on the other hand, is the thing that the scientist measures to test the effect of the treatment. These can be the same thing; such as when plants are exposed to different light treatments in a greenhouse and the dried weight of the whole plant is taken to assess how light levels affect biomass. If the dark and light halves of the greenhouse had 200 plants each, then there are a total of 400 experimental units (200 × 2 treatment levels) and 400 sampling units. However, if four leaves from each of the plants were measured instead to assess the response, then there would still be 400 experimental units (200 per treatment), but 1600 sampling units. Confounding experimental units and sampling units incorrectly in the statistical analysis can lead to accusations of pseudoreplication (see chapter 4 in Wiersma ( 2022a , b ) for a detailed discussion of this issue as it pertains to landscape experiments).
In this review, I examine experiments designed to understand ecological processes, where space is either an implicit or an explicit component of the study design. Most happen at the ‘typical’ landscape extents of 1–100 km, but I did not limit the review to such studies, since what a small organism perceives as a landscape may be a very small area of just a few square metres or centimetres. My focus is to examine as wide a range of experiments about ecological processes as possible to deduce trends and best practices. There is value to taking landscapes/space into consideration when studying ecological processes. Although many papers published in this journal have examined spatial dimensions of ecological processes (e.g., Webb et al. 2012 ; Ahmed et al. 2016 ; Paca et al. 2019 ; Sieger and Hovestadt 2021 ; Barik et al. 2022 ; Bedane et al. 2022 ; Datta et al. 2022 ; John et al. 2022 ), few of these have been explicitly experimental. Thus, this review examines landscape experiments on ecological processes and experiments on ecological processes carried out with a landscape ecology focus.
Box 1 Six approaches to landscape experiments (from Wiersma 2022a )
Large-scale manipulative experiments—these refer to landscape experiments at extents of ~ 15 ha or greater. These can be observational or manipulative. Examples of long-term manipulative experiments of this type include the Savannah River Experiment (Brinkerhoff et al. 2005 ), the Biological Dynamics of Forest Fragmentation Project (Bierregaard et al. 1992 ) and the Stability of Altered Forest Ecosystems (SAFE) Experiment (Ewers et al. 2011 ).
Experimental model landscapes—these refer to landscape experiment that manipulate a smaller area (usually on the order of 1–15 ha), usually in a more anthropogenically manipulated landscape, such as an agricultural field. Examples include the Bowling Green Fragmentation Experiment (With and Pavuk 2011 ) and the Kansas University Fragmentation Experiment (Holt et al. 1995 ).
Mesocosms—these refer to experiments in artificial containers (e.g., tanks, aquaria), which are either assembled by the researcher (pots with investigator controlled plants grown in them), or are subsets of natural systems (e.g., aquaria with water from an adjacent pond). The experimental design places the mesocosms in situ in the natural environment for experimentation (Srivistava et al. 2004 ).
Microcosms—like mesocosms, microcosms are container experiments; the difference here is that microcosms are naturally occurring containers, or habitats/ecosystems with delineated boundaries, for example pitcher plants or tank bromeliads (Srivistava et al. 2004 ).
In silico landscapes—this refers to experiments involving computer models. These could include (but are not limited to) statistical models, mathematical models, cellular automata and agent-based models.
Novel landscapes—Wiersma ( 2022a ) highlighted how experiments in non-terrestrial landscapes such as seascapes (Pittman 2018 ) and riverscapes (Wiens 2002 ) create opportunities for different kinds of experiments. Similarly, experiments that take a landscape ecology lens to other disciplines such as acoustic ecology (“soundscapes”; Farina 2014) or medical science (“tumor-scapes”; Lloyd et al. 2015 ) or construct artificial landscapes in a laboratory setting (“microlandscapes”; Larsen and Hargreaves 2020 ) can offer new opportunities for experiments to address questions in landscape ecology.
I searched the journal database Scopus (which indexes 18,000 titles from over 5000 international publishers) on 30-May-2022 for papers that addressed ecological process experiments at landscape extents. The search string TITLE-ABS-KEY (“ecological process”) AND TITLE-ABS-KEY (landscape) AND TITLE-ABS-KEY (experiment*) yielded 177 papers. After removing duplicates and government reports and those where I could not access the document (see Fig. 1 for summary), I reviewed the abstracts of all papers, and excluded review/essay/op-ed papers (31), methods papers (8), those with no explicit experiment (24), and those that did not examine an ecological process (14). This yielded 87 papers (see Additional file 1 for full list). For each paper, I noted whether the experiment was observational or manipulative and whether the experimental design was spatially implicit or explicit. I also noted the spatial extent of the study area (if this was not reported, I attempted to infer it either via estimation from included maps, or by searching the internet for details on the study area), the spatial extent of the treatment units (to calculate the scope; Frazier 2022 ; Wiersma and Schneider In press), the degree of replication (of both experimental units and study landscapes) and the type of ecological process under assessment.
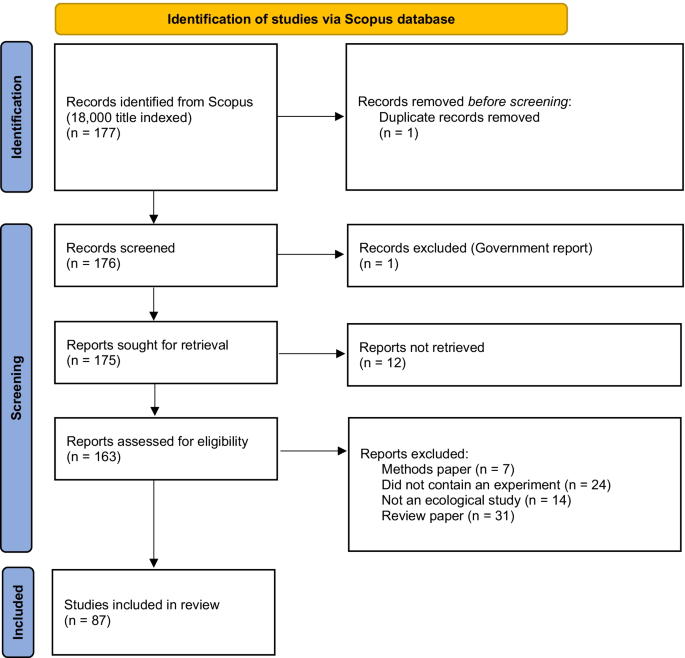
Schematic diagram of article identification and screening and sample size at each stage. Template for figure from: Page MJ, McKenzie JE, Bossuyt PM, Boutron I, Hoffmann TC, Mulrow CD, et al. The PRISMA 2020 statement: an updated guideline for reporting systematic reviews. BMJ 2021;372:n71. https://doi.org/10.1136/bmj.n71
Finally, I classified the experiment based on both type of question (following the taxonomy of Jenerette and Shen 2012 ) and by experimental method. For the latter, I used the six classes discussed in my book (Wiersma 2022a , b ) and summarized briefly in Wiersma ( 2022b ) and here in Box 1 . For clarity, definitions of meso- and microcosm here follow that in the book (Wiersma 2022a ), where mesocosms are artificial containers placed in the environment (e.g., tanks, aquaria) and microcosms are naturally occurring containers (e.g., pitcher plants, tank bromeliads). Microlandscapes refer to artificially constructed landscapes, which the experimenter manipulates under laboratory conditions (e.g., a dendritic network of pipes and petri dishes to assess ciliate movement). Because of the focus on ecological processes, I also noted a few other experiment types (e.g., food addition, seed addition, exclosures (to exclude predators/pollinators)) in addition to the categories in Wiersma ( 2022a , b ).
For the classification by experimental question, I tried to classify observational studies, even though Jenerette and Shen ( 2012 ) excluded such ‘natural experiments’ from their review. For example, I included observational studies that examined a natural disturbance as Type IV.13, even if the researcher did not actively manipulate the disturbance under study. Similarly, I classified observational studies that tested for differences in species distribution under different conditions as perception experiments (Type I.1), even if they were not explicitly manipulative. I did not classify the in silico experiments against Jenerette and Shen’s ( 2012 ) taxonomy, since their review focused on manipulative experiments.
Experiment types
The 87 papers reviewed represented a wide range of journals and disciplines (Table 1 ). Of these 87 papers, 15 were experiments in silico (discussed in further detail below). Of the remaining 72 papers, 17 were observational experiments, 45 were manipulative and 10 included a combination of an observational and manipulative experiment. Only one (Hess and Tschinkel 2017 ) used a full BACI (Before-After-Control-Impact) design. Three papers (Gornall et al. 2007 ; Lu et al. 2018 ; Menzies Pluer et al. 2020 ) had a lab component in addition to a field study, and one (Heggenes et al. 2017 ) transferred microcosms (lichen mats) from the field to the lab for the experimental treatment. The experimental methods are summarized in Table 2 . After in silico experiments, large-scale manipulative and large-scale observational experiments were most common (15 and 10 papers, respectively). As well, there were 11 experiments with some kind of addition, including food (6), artificial nests (1), seeds (2) and nutrients (2).
Research questions
The types of ecological processes addressed did not cover all the categories of Jenerette and Shen ( 2012 ); most common were manipulations of internal patch characteristics (Type III.7) and manipulation of disturbances (Type IV.13; although this count included natural disturbances; hence the number of observational studies in Table 3 does not match what is reported above.
Scale characteristics and replication
The spatial extent of the studies ranged from 78.5 m 2 to 20,300 km 2 and the size of the treatments from 4 cm 2 to ~ 500 km 2 for terrestrial studies, and 20 mL to 1000 L for aquatic/marine studies. The scope (ratio of extent to resolution/grain; Frazier 2022 ) ranged from 1.60 to 3.125 × 10 10 , with a mean of 4.17 × 10 8 . Variation in scope was narrowest for observational experiments and highest when studies combined both observational and manipulative experiments (Fig. 2 ). Replication of treatment units had a mean of 14.1 and median of 5 (range 1–320). Landscape replication was generally low, with 60 papers documenting an experiment in a single landscape, and only 7 papers documenting experiments in more than 2 landscapes (Marini 1997 ; Beckmann and Berger 2003 ; Cardoso et al. 2007 ; Hovel and Wahle 2010 ; Caballero-López et al. 2012 ; Bergerot et al. 2013 ; Augustine and Derner 2014 ; Giometto et al. 2014 ; Smith et al. 2014 ; Fronhofer and Altermatt 2015 ; Gillespie et al. 2017 ; Aristizábal and Metzger 2019 ; DiFiore et al. 2019 ; Menzies Pluer et al. 2020 ; Boone et al. 2022 ; Nunes and Byrne 2022 ).
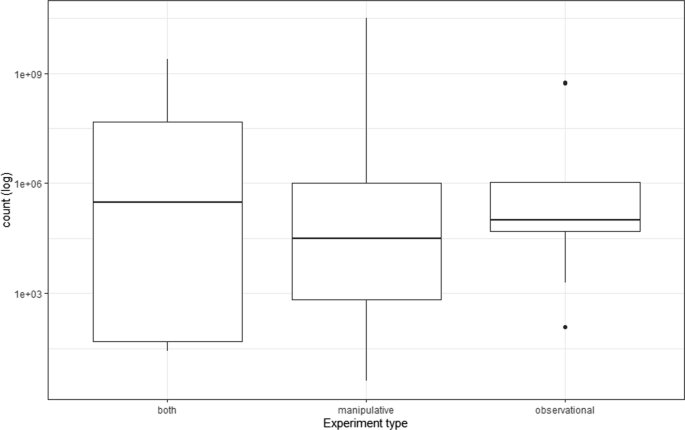
Scope (ratio of grain to extent) of 43 papers in this review. Note the log scale on the y -axis. Scope here is the minimum per paper; some papers had different grain sizes; only the smallest grain was used for the scope calculations. Note that data were not always available to calculate scope, and I only calculated scope for areal studies; studies incorporating treatments by volume or linear treatments (e.g., along streams, or soil depths) were excluded
Modelling tools
The in silico experiments used a range of modelling/computer tools, including cellular automata models (3), demographic models (1), agent-based models (1), process models (2), GIS/remote sensing (3), habitat models (2), scenario models (1) and mathematical models (2). Interestingly, the only paper in the collection obtained with the keyword search above to appear in this journal, was an in silico scenario model of the influence of ecological, economic and social drivers on future ecosystem goods and services (Huber et al. 2014 ). The majority of the in silico experiments modelled some kind of response to disturbance: either fire (Davies et al. 2021 ), grazing (King and Franz 2016 ; Verma et al. 2020 ), or climate change (Keane et al. 2017 ; Cui et al. 2021 ). Others modelled species movement (Samarasin et al. 2017 ; Baggio et al. 2019 ) or habitat use (Rowland et al. 2018 ; Muñoz et al. 2021 ) and still others modelled abiotic processes such as carbon (Güneralp et al. 2014 ; Xu et al. 2017 ), vegetation dynamics (Rango et al. 2002 ) or hydrology (Govind et al. 2011 ).
This review is an exploration of whether and how the themes of ecological processes, experiments and landscape ecology intersect. My findings suggests that experiments on ecological processes that have spatial dimensions occur in many kinds of ecological systems, including oceans (e.g., Cardoso et al. 2007 ), forests (e.g., Hylander 2005 ), urban areas (e.g., Visscher et al. 2018 ) and agricultural systems (e.g., Ouyang et al. 2020 ). Several of the experiments took place in long-term landscape-scale experimental sites, such as the Biological Dynamics of Forest Fragments Project (Laurance et al. 2002 ), the Savannah River Experiment (Levey et al. 2016 ), the Inner Mongolia Grassland Experiment (Yuan et al. 2015 ) and the Kansas Fragmentation Experiment (Alexander et al. 2012 ). Leveraging such long-term projects is a strategic approach to integrating landscape ecology perspectives into studies of ecological processes, since these sites have long-term data, as well as logistical resources and supports for researchers (Wiersma 2022a ).
The papers I reviewed examined a wide range of ecological processes, ranging from dispersal of organisms (e.g., Fronhofer and Altermatt 2015 ) or seeds (e.g., Miguel et al. 2018 ) to nutrient stocks and flows (e.g., Yuan et al. 2015 ). I also found papers carrying out experiments on species interactions such as pollination (e.g., Schmucki and De Blois 2009 ), predation (e.g., Gering and Blair 1999 ) and herbivory (e.g., DiFiore et al. 2019 ). A number of papers had an “applied” focus to restoration or management of ecological systems as evidenced by papers in the Journal of Applied Ecology (6), Restoration Ecology (2). Journal of Environmental Management (2), Forest Ecology and Management (2), Ecological Applications (1) and Ecological Management and Restoration (1) (Table 1 ).
Overall, it appears that many experiments concerned with ecological processes have taken a spatial/landscape approach, and at a range of extents and landscape types. This is not surprising, but what may be more surprising is the relatively low number (87 papers) of papers that are explicitly experimental. If we limit our characterization of an “experiment” to just manipulative experiments and exclude observational experiments, then this number drops to 55. Moreover, for a review focused on ecological processes, there was only a single paper from this journal (Huber et al. 2014 ); this documenting an in silico experiment. Although other papers in Ecological Processes are spatially explicit and borrow concepts and tools from landscape ecology, these did not appear in the keyword search, and were not presented by their authors as experiments. This is likely due to the challenges of doing robust experiments in landscape ecology (Jenerette and Shen 2012 ; Wiersma 2022a , b ).
Even where there are robust spatial experiments, as evidenced here, there can be challenges for researchers to meet the criteria of good experimental design. The majority of experiments occurred in a single landscape, thus making it difficult to assess if the inferences gained from one study would apply in a different landscape. This finding speaks, perhaps to the “case study” approach that characterized early work in landscape ecology (Opdam et al. 2002 ). Although case studies, whether qualitative or quantitative may not be fully replicable experiments, they certainly have a place in research; indeed in the medical and psychological fields, case studies are a major element of knowledge advancement (Stake 2008 ). Thus, researchers and reviewers should not dismiss case studies just because they may not be fully reproducible. Indeed, well-documented case studies can form the basis for valuable meta-analyses (Harrison 2011 ; Koricheva et al. 2013 ; Gerstner et al. 2017 ).
Where there was high replication (more than 2) of the experiment in different landscapes, this was often in anthropogenic systems, such as agricultural fields (Caballero-López et al. 2012 ; Augustine and Derner 2014 ), or when investigating dispersal of organisms that operate at smaller extents, such as butterflies (Bergerot et al. 2013 ) and ciliates (Giometto et al. 2014 ). A few were able to replicate landscapes across a broader extent, such as DiFiore et al. ( 2019 ), who examined two distinct coral reef system in the Caribbean. Experiments in this review generally had limited treatment replication; with 39 of the studies have 10 or fewer treatment replicates, and 25 having fewer than 5. All of the manipulative experiments had some kind of control; observational studies generally were comparisons in space and/or time and did not always have a strict control.
Overall, it appears that ecologists of all types and throughout the world are applying a great deal of creativity to experiments on ecological processes in landscapes. Most are meeting criteria of control and treatment replication; replication at landscape extents in more challenging, which is understandable. While many of the papers reviewed did not explicitly focus on landscape ecology, thinking about ecological process experiments in landscape context could yield useful insights. Experiments at smaller extents may be a strategic way to meet criteria of good experimental design, and with some effort, the inferences might be able to be scaled up to the extents at which human management happens. The experiment on soil organisms’ feeding activities by Joschko et al. ( 2008 ) is a good example of cross-scale work on ecological processes in a landscape. Since ecological processes are scaled in space and time, and landscape ecologists are familiar with scaling issues, considering how to extrapolate from small-scale process experiments to larger-extent landscapes is likely the next frontier to explore. A recent review by Wiersma and Schneider (In press) examined whether microlandscapes and sampling at small scales can usefully be extrapolated to make inferences at larger scales. Larsen and Hargreaves ( 2020 ) reviewed the broad array of microlandscape experiments, but did not examine scaling up in detail. Cross-scaling is facilitated when experiments are at different scales, but have the same scope; where scope is defined as the ratio of the extent to the grain (Frazier 2022 ). The scope of studies in this review varied several orders of magnitude (Fig. 2 ), making it difficult to compare across experiments. Ecologists considering experimental approaches as a means of understanding ecological processes in space would be wise to consider scale effects when designing the experiment. Whether experiments are manipulative or observational, researchers should make careful consideration of sampling design (including grain/extent, and hence scope), and degree of replication, randomization, experimental control and reproducibility in their studies. Although case studies have their place, experiments facilitate better understanding of the mechanisms influencing ecological processes, and thus should not be cast aside simply because they are difficult to do at landscape scales.
Availability of data and materials
The list of all papers reviewed is provided as an Excel spreadsheet in the additional material.
Ahmed F, Fakhruddin ANM, Imam MDT et al (2016) Spatial distribution and source identification of heavy metal pollution in roadside surface soil: a study of Dhaka Aricha highway, Bangladesh. Ecol Process 5:2. https://doi.org/10.1186/s13717-016-0045-5
Article Google Scholar
Alexander HM, Foster BL, Ballantyne F et al (2012) Metapopulations and metacommunities: combining spatial and temporal perspectives in plant ecology. J Ecol 100:88–103. https://doi.org/10.1111/j.1365-2745.2011.01917.x
Aristizábal N, Metzger JP (2019) Landscape structure regulates pest control provided by ants in sun coffee farms. J Appl Ecol 56:21–30. https://doi.org/10.1111/1365-2664.13283
Augustine DJ, Derner JD (2014) Controls over the strength and timing of fire-grazer interactions in a semi-arid rangeland. J Appl Ecol 51:242–250. https://doi.org/10.1111/1365-2664.12186
Baggio JA, Schoon ML, Valury S (2019) Managing networked landscapes: conservation in a fragmented, regionally connected world. Reg Environ Change 19:2551–2562. https://doi.org/10.1007/s10113-019-01567-8
Barik S, Saha GK, Mazumdar S (2022) Influence of land cover features on avian community and potential conservation priority areas for biodiversity at a Ramsar site in India. Ecol Process 11:1. https://doi.org/10.1186/s13717-022-00369-x
Beckmann JP, Berger J (2003) Using black bears to test ideal-free distribution models experimentally. J Mammal 84:594–606. https://doi.org/10.1644/1545-1542(2003)084%3c0594:UBBTTI%3e2.0.CO;2
Bedane GA, Feyisa GL, Senbeta F (2022) Spatial distribution of above ground carbon density in Harana Forest, Ethiopia. Ecol Process 11:4. https://doi.org/10.1186/s13717-021-00345-x
Bergerot B, Tournant P, Moussus J-P et al (2013) Coupling inter-patch movement models and landscape graph to assess functional connectivity. Popul Ecol 55:193–203. https://doi.org/10.1007/s10144-012-0349-y
Bierregaard RO, Lovejoy TE, Kapos V, Hutchings RW (1992) The biological dynamics of tropical rainforest fragments. Bioscience 42:859–866. https://doi.org/10.2307/1312085
Boone SR, Brehm AM, Mortelliti A (2022) Seed predation and dispersal by small mammals in a landscape of fear: effects of personality, predation risk and land-use change. Oikos. https://doi.org/10.1111/oik.08232
Brinkerhoff RJ, Haddad NM, Orrock JL (2005) Corridors and olfactory predator cues affect small mammal behavior. J Mammal 86:662–669. https://doi.org/10.1644/1545-1542(2005)086[0662:CAOPCA]2.0.CO;2
Caballero-López B, Bommarco R, Blanco-Moreno JM et al (2012) Aphids and their natural enemies are differently affected by habitat features at local and landscape scales. Biol Control 63:222–229. https://doi.org/10.1016/j.biocontrol.2012.03.012
Cardoso PG, Raffaelli D, Pardal MA (2007) Seagrass beds and intertidal invertebrates: an experimental test of the role of habitat structure. Hydrobiologia 575:221–230. https://doi.org/10.1007/s10750-006-0375-x
Cui F, Wang B, Zhang Q et al (2021) Climate change versus land-use change—what affects the ecosystem services more in the forest-steppe ecotone? Sci Total Environ 759:143525. https://doi.org/10.1016/j.scitotenv.2020.143525
Article CAS Google Scholar
Datta D, Bairagi M, Dey M et al (2022) Spatially explicit estimation of soil organic carbon stock of an estuarine mangrove wetland of eastern India using elemental analysis and very-fine resolution satellite data. Ecol Process 11:30. https://doi.org/10.1186/s13717-022-00370-4
Davies HF, Visintin C, Gillespie GR, Murphy BP (2021) Investigating the effects of fire management on savanna biodiversity with grid-based spatially explicit population simulations. J Appl Ecol 58:677–686. https://doi.org/10.1111/1365-2664.13801
Diamond JM (1983) Ecology: laboratory, field and natural experiments. Nature 304:586–587. https://doi.org/10.1038/304586a0
Diamond J (2001) Ecology: dammed experiments! Science 294:1847–1848. https://doi.org/10.1126/science.1067012
DiFiore BP, Queenborough SA, Madin EMP et al (2019) Grazing halos on coral reefs: predation risk, herbivore density, and habitat size influence grazing patterns that are visible from space. Mar Ecol Prog Ser 627:71–81. https://doi.org/10.3354/meps13074
Ewers RM, Didham RK, Fahrig L et al (2011) A large-scale forest fragmentation experiment: the stability of altered forest ecosystems project. Phil Trans Royal Soc B Biol Sci 366:3292–3302. https://doi.org/10.1098/rstb.2011.0049
Frazier AE (2022) Scope and its role in advancing a science of scaling in landscape ecology. Landsc Ecol. https://doi.org/10.1007/s10980-022-01403-1
Fronhofer EA, Altermatt F (2015) Eco-evolutionary feedbacks during experimental range expansions. Nat Commun 6:6844. https://doi.org/10.1038/ncomms7844
Gering JC, Blair RB (1999) Predation on artificial bird nests along an urban gradient: predatory risk or relaxation in urban environments? Ecography 22:532–541. https://doi.org/10.1111/j.1600-0587.1999.tb00542.x
Gerstner K, Moreno-Mateos D, Gurevitch J et al (2017) Will your paper be used in a meta-analysis? Make the reach of your research broader and longer lasting. Methods Ecol Evol 8:777–784. https://doi.org/10.1111/2041-210X.12758
Gillespie MAK, Baude M, Biesmeijer J et al (2017) A method for the objective selection of landscape-scale study regions and sites at the national level. Methods Ecol Evol 8:1468–1476. https://doi.org/10.1111/2041-210X.12779
Giometto A, Rinaldo A, Carrara F, Altermatt F (2014) Emerging predictable features of replicated biological invasion fronts. Proc Natl Acad Sci USA 111:297–301. https://doi.org/10.1073/pnas.1321167110
Gornall JL, Jónsdóttir IS, Woodin SJ, Van Der Wal R (2007) Arctic mosses govern below-ground environment and ecosystem processes. Oecologia 153:931–941. https://doi.org/10.1007/s00442-007-0785-0
Govind A, Chen JM, Mcdonnell J et al (2011) Effects of lateral hydrological processes on photosynthesis and evapotranspiration in a boreal ecosystem. Ecohydrology 4:394–410. https://doi.org/10.1002/eco.141
Güneralp I, Filippi AM, Randall J (2014) Estimation of floodplain aboveground biomass using multispectral remote sensing and nonparametric modeling. Int J Appl Earth Obs Geoinf 33:119–126. https://doi.org/10.1016/j.jag.2014.05.004
Haddad NM (2012) Connecting ecology and conservation through experiment. Nat Methods 9:794–795. https://doi.org/10.1038/nmeth.2107
Harrison F (2011) Getting started with meta-analysis. Methods Ecol Evol 2:1–10. https://doi.org/10.1111/j.2041-210X.2010.00056.x
Heggenes J, Odland A, Chevalier T et al (2017) Herbivore grazing—or trampling? Trampling effects by a large ungulate in cold high-latitude ecosystems. Ecol Evol 7:6423–6431. https://doi.org/10.1002/ece3.3130
Hess CA, Tschinkel WR (2017) Effect of thinning and clear-cuts on the transmission of fire in slash pine plantations during restoration to longleaf pine. Ecol Restor 35:33–40. https://doi.org/10.3368/er.35.1.33
Holt RD, Robinson GR, Gaines MS (1995) Vegetation dynamics in an experimentally fragmented landscape. Ecology 76:1610–1624. https://doi.org/10.2307/1938162
Hovel KA, Wahle RA (2010) Effects of habitat patchiness on American lobster movement across a gradient of predation risk and shelter competition. Ecology 91:1993–2002. https://doi.org/10.1890/09-0595.1
Huber R, Briner S, Bugmann H et al (2014) Inter- and transdisciplinary perspective on the integration of ecological processes into ecosystem services analysis in a mountain region. Ecol Process 3:9. https://doi.org/10.1186/2192-1709-3-9
Hylander K (2005) Aspect modifies the magnitude of edge effects on bryophyte growth in boreal forests. J Appl Ecol 42:518–525. https://doi.org/10.1111/j.1365-2664.2005.01033.x
Jenerette GD, Shen W (2012) Experimental landscape ecology. Landsc Ecol 27:1237–1248. https://doi.org/10.1007/s10980-012-9797-1
John K, Bouslihim Y, Isong IA et al (2022) Mapping soil nutrients via different covariates combinations: theory and an example from Morocco. Ecol Process 11:23. https://doi.org/10.1186/s13717-022-00368-y
Joschko M, Oehley J, Gebbers R et al (2008) A spatial approach to soil-ecological experimentation at landscape scale. J Plant Nutr Soil Sci 171:338–343. https://doi.org/10.1002/jpln.200700088
Keane RE, Holsinger LM, Mahalovich MF, Tomback DF (2017) Evaluating future success of whitebark pine ecosystem restoration under climate change using simulation modeling. Restor Ecol 25:220–233. https://doi.org/10.1111/rec.12419
King EG, Franz TE (2016) Combining ecohydrologic and transition probability-based modeling to simulate vegetation dynamics in a semi-arid rangeland. Ecol Model 329:41–63. https://doi.org/10.1016/j.ecolmodel.2016.02.019
Kohler RE (2002) Labscapes and landscapes: exploring the lab-field border in biology. University of Chicago Presss, Chicago and London
Book Google Scholar
Koricheva J, Gurevitch J, Mengersen K (eds) (2013) Handbook of meta-analysis in ecology and evolution. Princeton University Press, Princeton
Google Scholar
Krebs CJ (1989) Ecological methodology. Harper Collins, New York
Larsen C, Hargreaves A (2020) Miniaturizing landscapes to understand species distributions. Ecogrpahy 43:1625–1638. https://doi.org/10.1111/ecog.04959
Laurance WF, Lovejoy TE, Vasconcelos HL et al (2002) Ecosystem decay of Amazonian forest fragments: a 22-year investigation. Conserv Biol 16:605–618. https://doi.org/10.1046/j.1523-1739.2002.01025.x
Levey DJ, Caughlin TT, Brudvig LA et al (2016) Disentangling fragmentation effects on herbivory in understory plants of longleaf pine savanna. Ecology 97:2248–2258. https://doi.org/10.1002/ecy.1466
Lloyd MC, Rejniak KA, Brown JS et al (2015) Pathology to enhance precision medicine in oncology: lessons from landscape ecology. Adv Anat Pathol 22:267–272. https://doi.org/10.1097/PAP.0000000000000078
Lu W, Xiao J, Lei W et al (2018) Human activities accelerated the degradation of saline seepweed red beaches by amplifying top-down and bottom-up forces. Ecosphere 9:e02352. https://doi.org/10.1002/ecs2.2352
Marini MA (1997) Predation-mediated bird nest diversity: an experimental test. Can J Zool 75:317–323. https://doi.org/10.1139/z97-040
Menzies Pluer EG, Schneider RL, Morreale SJ et al (2020) Returning degraded soils to productivity: an examination of the potential of coarse woody amendments for improved water retention and nutrient holding capacity. Water Air Soil Pollut 231:15. https://doi.org/10.1007/s11270-019-4380-x
Miguel MF, Tabeni S, Cona MI, Campos CM (2018) Secondary seed dispersal by mammals between protected and grazed semiarid woodland. For Ecol Manage 422:41–48. https://doi.org/10.1016/j.foreco.2018.03.056
Muñoz P, García-Rodríguez A, Sandoval L (2021) Urbanization, habitat extension and spatial pattern, threaten a costa Rican endemic bird. Revista De Biologia Trop 69:170–180. https://doi.org/10.15517/RBT.V69I1.41742
Nunes AM, Byrne KM (2022) Drought and shrub cover differentially affect seed bank composition within two sagebrush steppe communities. J Arid Environ 202:104752. https://doi.org/10.1016/j.jaridenv.2022.104752
Opdam P, Foppen R, Vos C (2002) Bridging the gap between ecology and spatial planning in landscape ecology. Landsc Ecol 16:767–779
Ouyang F, Su W, Zhang Y et al (2020) Ecological control service of the predatory natural enemy and its maintaining mechanism in rotation-intercropping ecosystem via wheat-maize-cotton. Agr Ecosyst Environ 301:107024. https://doi.org/10.1016/j.agee.2020.107024
Paca VHM, Espinoza-Dávalos GE, Hessels TM et al (2019) The spatial variability of actual evapotranspiration across the Amazon River Basin based on remote sensing products validated with flux towers. Ecol Process 8:6. https://doi.org/10.1186/s13717-019-0158-8
Pittman SJ, Wiens JA, Wu J, Urban DL (2018) Landscape ecologists’ perspectives on seascape ecology. In: Pittman SJ (ed) Seascape ecology. Wiley Blackwell, Hoboken, pp 485–492
Rango A, Goslee S, Herrick J et al (2002) Remote sensing documentation of historic rangeland remediation treatments in southern New Mexico. J Arid Environ 50:549–572. https://doi.org/10.1006/jare.2001.0865
Rowland MM, Wisdom MJ, Nielson RM et al (2018) Modeling elk nutrition and habitat use in western Oregon and Washington. Wildl Monogr 199:1–6
Samarasin P, Shuter BJ, Wright SI, Rodd FH (2017) The problem of estimating recent genetic connectivity in a changing world. Conserv Biol 31:126–135. https://doi.org/10.1111/cobi.12765
Schmucki R, De Blois S (2009) Pollination and reproduction of a self-incompatible forest herb in hedgerow corridors and forest patches. Oecologia 160:721–733. https://doi.org/10.1007/s00442-009-1347-4
Sieger CS, Hovestadt T (2021) The effect of landscape structure on the evolution of two alternative dispersal strategies. Ecol Process 10:73. https://doi.org/10.1186/s13717-021-00343-z
Smith AL, Bull CM, Gardner MG, Driscoll DA (2014) Life history influences how fire affects genetic diversity in two lizard species. Mol Ecol 23:2428–2441. https://doi.org/10.1111/mec.12757
Srivastava DS, Kolasa J, Bengtsson J et al (2004) Are natural microcosms useful model systems for ecology? Trends Ecol Evol 19:379–384. https://doi.org/10.1016/j.tree.2004.04.010
Stake RE (2008) Qualitative case studies. Strategies of qualitative inquiry. Sage Publications, Thousand Oaks, pp 119–149
Turner MG (2005) Landscape ecology in North America: past, present, and future. Ecology 86:1967–1974. https://doi.org/10.1890/04-0890
Turner MG, Gardner RH (2015) Landscape ecology in theory and practice: pattern and process. Springer-Verlag, New York
Verma M, Schulte to Bühne H, Lopes M et al (2020) Can reindeer husbandry management slow down the shrubification of the Arctic? J Environ Manage 267:110636. https://doi.org/10.1016/j.jenvman.2020.110636
Visscher DR, Unger A, Grobbelaar H, Dewitt PD (2018) Bird foraging is influenced by both risk and connectivity in urban parks. J Urban Ecol 4:juy020. https://doi.org/10.1093/jue/juy0020
Webb SL, Olson CV, Dzialak MR et al (2012) Landscape features and weather influence nest survival of a ground-nesting bird of conservation concern, the greater sage-grouse, in human-altered environments. Ecol Process 1:4. https://doi.org/10.1186/2192-1709-1-4
Wiens JA (2002) Riverine landscapes: taking landscape ecology into the water. Freshw Biol 47:501–515. https://doi.org/10.1046/j.1365-2427.2002.00887.x
Wiersma YF (2022a) Experimental landscape ecology. Springer, New York
Wiersma YF (2022b) Carrying out experiments in landscape ecology. Landscape Ecol 37:1729–1732. https://doi.org/10.1007/s10980-022-01459-z
Wiersma YF, Schneider DC (In press) Microlandscape experiments: are they useful for scale, scaling, and cross-scale inference? Curr Landsc Ecol Rep
With KA, Pavuk DM (2011) Habitat area trumps fragmentation effects on arthropods in an experimental landscape system. Landscape Ecol 26:1035–1048. https://doi.org/10.1007/s10980-011-9627-x
Xu B, Pan Y, Plante AF et al (2017) Modeling forest carbon cycle using long-term carbon stock field measurement in the Delaware River Basin. Ecosphere 8:e01802. https://doi.org/10.1002/ecs2.1802
Yuan F, Wu J, Li A et al (2015) Spatial patterns of soil nutrients, plant diversity, and aboveground biomass in the Inner Mongolia grassland: before and after a biodiversity removal experiment. Landscape Ecol 30:1737–1750. https://doi.org/10.1007/s10980-015-0154-z
Download references
Acknowledgements
Thank you to Jiquan Chen for the invitation to submit this review paper.
There was no funding received from any funding sources for this publication.
Author information
Authors and affiliations.
Department of Biology, Memorial University, St. John’s, NL, A1C 5S7, Canada
Yolanda F. Wiersma
You can also search for this author in PubMed Google Scholar
Contributions
YFW collected and reviewed the articles, wrote and revised the paper. The author read and approved the final manuscript.
Corresponding author
Correspondence to Yolanda F. Wiersma .
Ethics declarations
Ethics approval and consent to participate.
Not applicable.
Consent for publication
Competing interests.
The author declares that they have no financial and non-financial competing interests.
Additional information
Publisher's note.
Springer Nature remains neutral with regard to jurisdictional claims in published maps and institutional affiliations.
Supplementary Information
Additional file 1..
The list of all papers reviewed is provided as an Excel spreadsheet.
Rights and permissions
Open Access This article is licensed under a Creative Commons Attribution 4.0 International License, which permits use, sharing, adaptation, distribution and reproduction in any medium or format, as long as you give appropriate credit to the original author(s) and the source, provide a link to the Creative Commons licence, and indicate if changes were made. The images or other third party material in this article are included in the article's Creative Commons licence, unless indicated otherwise in a credit line to the material. If material is not included in the article's Creative Commons licence and your intended use is not permitted by statutory regulation or exceeds the permitted use, you will need to obtain permission directly from the copyright holder. To view a copy of this licence, visit http://creativecommons.org/licenses/by/4.0/ .
Reprints and permissions
About this article
Cite this article.
Wiersma, Y.F. A review of landscape ecology experiments to understand ecological processes. Ecol Process 11 , 57 (2022). https://doi.org/10.1186/s13717-022-00401-0
Download citation
Received : 26 July 2022
Accepted : 26 August 2022
Published : 09 September 2022
DOI : https://doi.org/10.1186/s13717-022-00401-0
Share this article
Anyone you share the following link with will be able to read this content:
Sorry, a shareable link is not currently available for this article.
Provided by the Springer Nature SharedIt content-sharing initiative
- Landscape ecology
- Manipulative experiment
- Observational experiment

IMAGES
VIDEO
COMMENTS
Ecological methods for research entail observing, experimenting and modeling. There are many subtypes of these overarching methods. Observation and field work are used to collect data. Manipulative, natural or observation experiments may be used. Modeling helps analyze the collected data.
What are types of ecological studies? Ecological studies can involve both laboratory or field research that examine the biological and physical processes involved in habitat health and diversity.
Ecological study. In epidemiology, ecological studies are used to understand the relationship between outcome and exposure at a population level, where 'population' represents a group of individuals with a shared characteristic such as geography, ethnicity, socio-economic status of employment. [1] What differentiates ecological studies from ...
Ecological research refers to the study of social-ecological-evolutionary processes and their potential contributions to environmental justice, with the aim of reducing racial and social disparities in environmental provisioning and improving global environmental sustainability. AI generated definition based on: Trends in Ecology & Evolution, 2021.
Scientific Method for Ecological Research. This Book has been cited by the following publications. This list is generated based on data provided by. VISUAL DEBUGGING: A WAY OF ANALYZING, UNDERSTANDING AND COMMUNICATING BOTTOM‐UP SIMULATION MODELS IN ECOLOGY. Natural Resource Modeling, Vol. 15, Issue. 1, p.
An ecological study is defined as a type of group-based study that compares the mean values of exposures and outcomes of different population groups, providing important insights for population health monitoring and hypothesis generation in social sciences.
The outcome is a text that provides a framework for understanding methodological issues and which assists with the effective definition and planning of ecological research. As such, it represents a unique resource for anyone embarking on their research career.
An ecological study is an observational study in which at least one variable is measured at the group level. An ecological study is especially appropriate for the initial investigation of causal hypotheses.
An ecological system can be a single organism and its surroundings, a population or set of interacting populations in a certain habitat, or the entire community together with the abiotic environment with which these species interact, a unit termed an ecosystem. Ecologists are interested both in the structure and function of ecological systems.
Overview Ecological Research is the official English language journal of The Ecological Society of Japan launched in 1986. The journal publishes original research papers, reviews, technical reports, notes and comments, and data papers covering all aspects of ecology and ecological sciences.
Here, we ask if these changes are reflected in actual ecological research over the last 30 years. We surveyed 750 articles from the entire pool of ecological literature and 750 articles from eight leading journals. Each article was characterized according to its type, ecological domain, and applicability, and major topics.
Learn about the advantages and disadvantages of ecological studies, a type of correlational research that examines population-level data. Find examples and applications from Boston University School of Public Health.
Discover how ecological research themes have changed over four decades in this journal article from Frontiers in Ecology and the Environment.
Summary Fundamental ecological research is both intrinsically interesting and provides the basic knowledge required to answer applied questions of importance to the management of the natural world. The 100th anniversary of the British Ecological Society in 2013 is an opportune moment to reflect on the current status of ecology as a science and look forward to high-light priorities for future ...
This Perspective discusses using long-term ecological research networks to advance our understanding of fundamental evolutionary processes and evolutionary responses to global change.
An ecological study is an observational study that is characterized by trying to relate exposure and disease at the region and population level, rather than at the individual level. •. Ecological studies are often used to measure prevalence and incidence of disease, particularly when disease is rare.
Ecological Research will be published in online-only format effective with the 2024 volume. This is a proactive move towards reducing the environmental impact caused by the production and distribution of printed journal copies and will allow the journal to invest in further innovation, digital development, and sustainability measures.
The increasing use of quantitative research synthesis in ecological and environmental sciences 19, 20 makes the consequences of biases in empirical studies especially severe, because the effects ...
Chapter 6. Ecological studies. More chapters in Epidemiology for the uninitiated. Most epidemiological investigations of aetiology are observational. They look for associations between the occurrence of disease and exposure to known or suspected causes. In ecological studies the unit of observation is the population or community.
This paper introduces research findings from a mixed methods study that used an ecological approach when investigating student transitions between a formal lecture theatre and adjacent informal ...
Specifically, this review seeks to discover how scientists design experiments to understand ecological processes at landscape scales. I found 87 papers where these three concepts intersected, and reviewed them in more depth to assess characteristics of scale (treatment and study area extent), replication, research question and experiment type.
The impacts of plastic pollution on soil ecosystems have emerged as a significant global environmental concern. The progress in understanding how plastic pollution affects soil microbial ...
Granular activated carbon (GAC) adsorption is frequently used to remove recalcitrant organic micropollutants (MPs) from water. The overarching aim of this research was to develop machine learning (ML) models to predict GAC performance from adsorbent, adsorbate, and background water matrix properties. For model calibration, MP breakthrough curves were compiled and analyzed to determine the bed ...